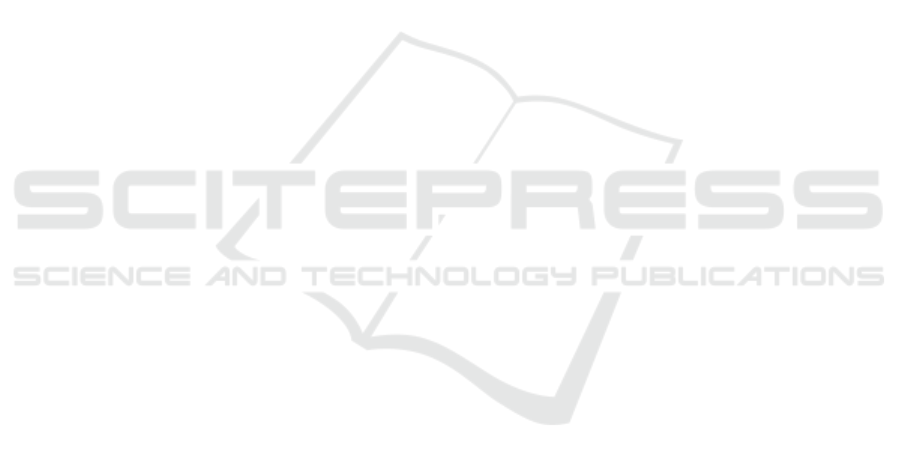
4 CONCLUSIONS
With regard to study aims, in these initial findings we
conclude:
a) Digital measurements with Kinect are not
appropriate for clinical trials demanding
high precision. There is no statistical
evidence that could differentiate distances of
examinee from Kinect sensor in order to
define optimal distance (as long as subject
stands within Kinect’s range)
b) Recommended number of measurements
with Kinect is 6,
c) Reliability of Kinect is excellent for height
and acceptable for left forearm length and
left lower leg length, and
d) Small errors occur due to clothing, possibly
due to illumination, and sensor height and
distance, which is in line with previous
research (e.g. Espitia et al., 2015)
For improving digital measurement of human
body it is advisable to:
1. Determine correction factors for further
reduction of measurement error,
2. Determine metric characteristics for Kinect
using other anthropometric measurements,
3. Standardize protocols for Kinect
measurements with regard to specific
environment conditions (e.g. indoor vs
outdoor), and
4. Include gender differentiators within a larger
sample in order to generalize phenomena
with better accuracy.
ACKNOWLEDGEMENTS
Research was conducted by joint Research Group of
Laboratory for Sport Medicine & Exercise -
Kinantropometry and Biomechanics Laboratory of
the Institute of Kinesiology, Faculty of Kinesiology,
as a part of joint IRCRO project “Development of a
Computer System for Digital Measurements of the
Human Body”, between the Faculty of Kinesiology
and companies Live Good j.d.o.o. and CITUS d.o.o.
Authors declare that there is no conflict of interest.
REFERENCES
Boonbrahm, P., Sewata, L., & Boonbrahm, S. (2015).
Transforming 2D Human Data into 3D Model for
Augmented Reality Applications. Procedia Computer
Science, 75(Vare), 28–33. http://doi.org/10.1016/
j.procs.2015.12.193
Cippitelli, E., Gasparrini, S., Spinsante, S., & Gambi, E.
(2015). Kinect as a tool for gait analysis: validation of
a real-time joint extraction algorithm working in side
view. Sensors (Basel, Switzerland), 15(1), 1417–1434.
http://doi.org/10.3390/s150101417
Clarkson, S., Wheat, J., Heller, B., & Choppin, S. (2016).
Assessment of a Microsoft Kinect-based 3D scanning
system for taking body segment girth measurements: a
comparison to ISAK and ISO standards. Journal of
Sports Sciences, 34(11), 1006–14. http://doi.org/
10.1080/02640414.2015.1085075
Clarkson, Sean, Wheat, Jonathan, Heller, Ben and Choppin,
Simon (2014). Assessing the suitability of the
Microsoft Kinect for calculating person specific body
segment parameters. In: 4th IEEE Workshop on
Consumer Depth Cameras for Computer Vision,
Zurich, Switzerland, 6th September 2014.
De Rosario, H., Belda-Lois, J. M., Fos, F., Medina, E.,
Poveda-Puente, R., & Kroll, M. (2014). Correction of
joint angles from kinect for balance exercising and
assessment. Journal of Applied Biomechanics, 30(2),
294–299. http://doi.org/10.1123/jab.2013-0062
Dutta, A., Chugh, S., Banerjee, A., & Dutta, A. (2014).
Point-of-care-testing of standing posture with Wii
balance board and microsoft kinect during transcranial
direct current stimulation: A feasibility study.
NeuroRehabilitation, 34(4), 789–798. http://doi.org/
10.3233/NRE-141077
Espitia- Contreras A., Sanchez-Caiman P., Uribe-Quevedo
A., (2015) Development of Kinect-base
Anthropometric Measuremnt Application. Nueva
Grenada Mil.University, Industrial Engineering
Galna, B., Jackson, D., Schofield, G., McNaney, R.,
Webster, M., Barry, G., … Rochester, L. (2014).
Retraining function in people with Parkinson’s disease
using the Microsoft kinect: Game design and pilot
testing. Journal of NeuroEngineering and
Rehabilitation, 11(1), 1–12. http://doi.org/10.1186/
1743-0003-11-60
Gao, Z., Yu, Y., Zhou, Y., & Du, S. (2015). Leveraging two
kinect sensors for accurate full-body motion capture.
Sensors (Switzerland), 15(9), 24297–24317. http://
doi.org/10.3390/s150924297
Gasparrini, S., Cippitelli, E., Spinsante, S., & Gambi, E.
(2014). A depth-based fall detection system using a
Kinect® sensor. Sensors (Basel, Switzerland), 14(2),
2756–75. http://doi.org/10.3390/s140202756
Lim, D., Kim, C., Jung, H., Jung, D., & Chun, K. (2015).
Use of the microsoft kinect system to characterize
balance ability during balance training. Clinical
Interventions in Aging, 10, 1077–1083. http://doi.org/
10.2147/CIA.S85299
Lun, R., & Zhao, W. (2015). A Survey of Applications and
Human Motion Recognition with Microsoft Kinect.
International Journal of Pattern Recognition and
Artificial Intelligence (Vol. 29). http://doi.org/10.1142/
S0218001415550083
icSPORTS 2016 - 4th International Congress on Sport Sciences Research and Technology Support
152