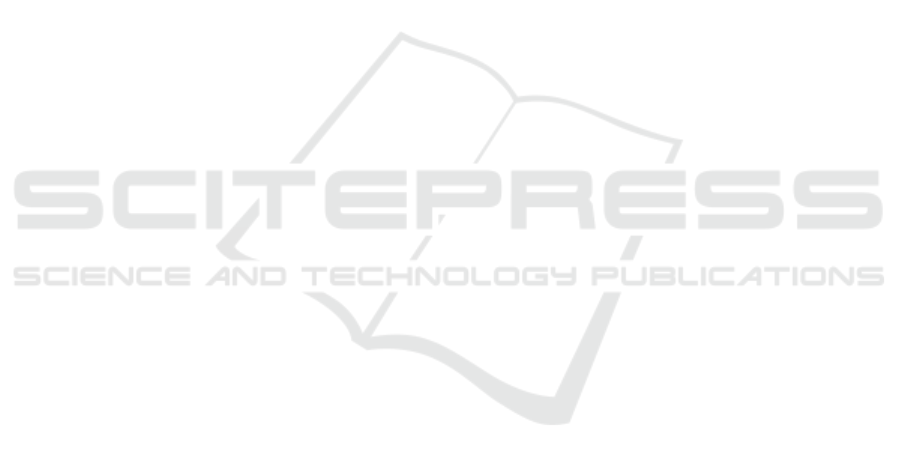
operation and to test in advance the prosthesis against
the patient-specific 3D reproduction of the knee joint
articulation (the KA). In so doing, a relevant amount
of time can be saved for settings and measurements,
and the KA can help reduce the operation time,
improve safety and lead to better outcome.
As purported in the specialist literature and
confirmed in our observational studies, 3DP has got a
potential to change the work of surgeons, both in
regard to surgery planning, and in educational
activities with novices, as well as in the
communication with the patient. In this sense,
medical 3DP objects represent a new toolkit of KAs
available to prosthetic practitioners, as these artifacts
allow for the patient-specific configuration and
setting of the main parameters and measurements that
can be tested before the surgery takes place. In this
light, further research should be aimed at
understanding whether prototype replicas can help
practitioners replace the more traditional “diagnostics
by imaging” paradigm with a complementary, if not
alternative, one: a “diagnostics by volumes”, which
would enable the emergence of new knowledge
circulation practices and habits.
ACKNOWLEDGEMENTS
This article has been developed under the DiDIY
project funded from the European Union’s Horizon
2020 research and innovation programme under grant
agreement No 644344.
REFERENCES
Auricchio, F., & Marconi, S., 2016. 3D printing: clinical
applications in orthopaedics and traumatology. EFORT
Open Reviews, 1(5), 121-127.
Bull, G., & Garofalo, J., 2009. Personal Fabrication
Systems: From Bits to Atoms. Learning & Leading with
Technology, 36(7), 10-12.
Cabitza, F., Colombo, G., & Simone, C. 2013. Leveraging
underspecification in knowledge artifacts to foster
collaborative activities in professional communities. Int
J Hum-Comput St, 71(1), 24-45.
Cabitza, F., Fogli, D., & Locoro, A., 2016. Virtual patients
for knowledge sharing and clinical practice training: a
gamified approach. GALA 2015, LNCS, 329-335.
Cabitza, F., Locoro, A., 2014a. Made with knowledge:
Disentangling the IT Knowledge Artifact by a
qualitative literature review. In KMIS 2014, 64-75.
Cabitza, F., Cerroni, A., Locoro, A., Simone, C. 2014b. The
knowledge-stream model: A comprehensive model for
knowledge circulation in communities of
knowledgeable practitioners. In KMIS 2014, 367-374.
Cabitza, F., Cerroni, A., & Simone, C. 2014c. Knowledge
artifacts within knowing communities to foster
collective knowledge. In AVI 2014, ACM, 391-394.
Cuendet, S., Bumbacher, E., and Dillenbourg, P. 2012.
Tangible vs. virtual representations: when tangibles
benefit the training of spatial skills. In NordiCHI '12.
de Mel, A., 2016. Three-dimensional printing and the
surgeon. British Journal of Surgery, 103(7), 786-788.
DiDIY, D3.1-1.2, Deliverable Doc no 3.1 of the DiDIY
project, Sept 2016.
Dourish, P. 2001. Where the Action is: The Foundations of
Embodied Interaction. MIT Press, Cambridge, USA.
Eltorai, A. E., Nguyen, E., & Daniels, A. H. 2015. Three-
Dimensional Printing in Orthopedic Surgery.
Orthopedics, 38(11), 684-687.
Fedorov A., Beichel R., Kalpathy-Cramer J., … Kikinis R.,
2012. 3D Slicer as an Image Computing Platform for
the Quantitative Imaging Network. Magnetic
Resonance Imaging, 30(9), 1323-41.
Greenhalgh, T., & Wieringa, S., 2011. Is it time to drop the
‘knowledge translation’metaphor? A critical literature
review. Journal of the Royal Society of Medicine,
104(12), 501-509.
Hornecker, E. & Buur, J. 2006. Getting a grip on tangible
interaction: a framework on physical space and social
interaction. In: Procs of ACM CHI 2006. 437-446.
Lakoff, G., & Johnson, M. 1999. Philosophy in the flesh:
The embodied mind and its challenge to western
thought. Basic books.
Latour, B., 2004. Why has critique run out of steam? From
matters of fact to matters of concern. Critical inquiry
30(2), 225-248.
Malik, H. H., Darwood, A., … & Baskaradas, A., 2015.
Three-dimensional printing in surgery: a review of
current surgical applications. Journal of Surgical
Research, 199(2), 512-522.
McMenamin, P. G., Quayle, M. R., McHenry, C. R., &
Adams, J. W., 2014. The production of anatomical
teaching resources using three-dimensional (3D)
printing technology. Anatomical sciences education,
7(6), 479-486.
Mitsouras, D., Liacouras, P., ... & Ho, V. B., 2015. Medical
3D printing for the radiologist. RadioGraphics, 35(7),
1965-1988.
Mok, S. W., Nizak, R., ... & Malda, J., 2016. From the
printer: Potential of three-dimensional printing for
orthopaedic applications. Journal of Orthopaedic
Translation, 6, 42-49.
Pernin, J. P., Michau, F., Mandran, N., & Mariais, C. 2012.
ScenLRPG, a board game for the collaborative design
of GBL scenarios: qualitative analysis of an
experiment. In Proc. of the 6th European Conference
on Games Based Learning, 384-392.
Popescu, D., Laptoiu, D., Hadar, A., Ilie, C., & Pârvu, C.,
2015. Workflow for additive manufacturing of an
individualized surgical template. Procs in
Manufacturing Systems, 10(3), 131-140.
More Time for the Doing, Having Made the Thinking - 3D Printing for Knowledge Circulation in Healthcare
297