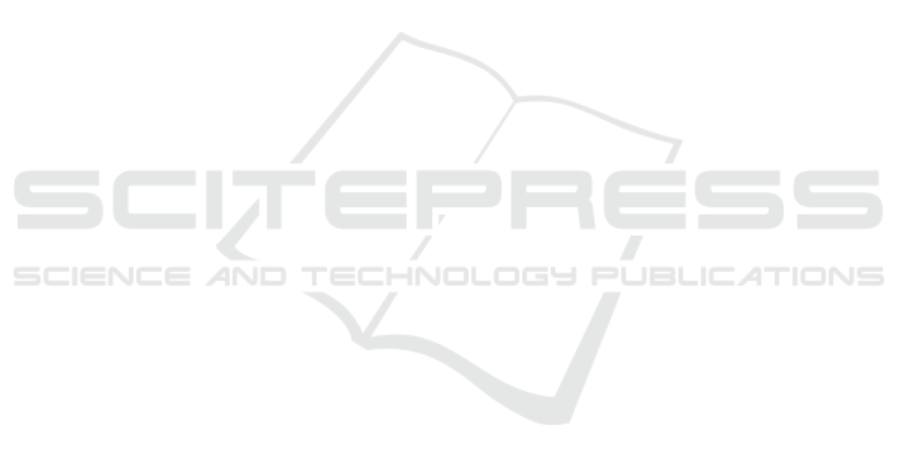
access point. In reception, the received packets are
checked, treated and send to interior tiles by the mesh
routers. Provided converter technology by our partner
NXP envisions a 20 GHz bandwidth for the system.
Based on the design constraints and circuit
simulations, most suitable spectrum is chosen
between 20-40 GHz (Hamieh et al., 2014).
It is decided to have 1024 subcarriers, thus 1024-
point FFT and IFFT blocks are required. Hence, as we
have a 20 GHz bandwidth with 1024 subcarriers, we
have subcarrier frequency spacing of 19.53 MHz,
where a symbol duration is T = 1=19.53 MHz = 51.2
ns. Fig. 2, illustrates the architecture of the RF front-
ends. The up-conversion mixers combine a baseband
signal with a local oscillator signal. Mixing occurs in
a MOSFET, whose gate and drain are respectively fed
by the local oscillator and the baseband signal. As the
local oscillator frequency is 30 GHz, which is the
middle of our 20 GHz bandwidth, it needs to be
suppressed. Thanks to the differential outputs of the
DAC, two IQ-Modulators can work together to do so.
Besides avoiding interference caused by image
frequencies they can reduce the LO level in the
output. As we use the same local oscillator for both of
IQ-modulators and opposite I-Q signals, the IQ-
Modulators outputs are subtracted in a differential
amplifier to perform this suppression. Then this
signal is amplified by a Low-Noise Amplifier (LNA)
and transmitted on waveguide.
The reception is done synchronously every T =
51.2 ns as transmission, too. The received signal from
the transmission line is amplified and fed to a
separator circuit, mixers and 30 GHz local oscillator
to obtain in-phase and quadrature components. Low
Pass Filters (LPF) are used for down-conversion.
Then I and Q components are converted to digital
domain by our Analog-to-Digital (ADC)
components. After Serial to Parallel conversion this
vector of I and Q values are converted to frequency
domain by an FFT block. Utilized FFT/IFFT
processors are estimated to be manufactured with 120
nm CMOS technology. We estimate the area of each
of these modules as 0.31 mm
2
and power consumption
of 67.5 mW. Each of ADCs and DACs are designed
with 120 nm technology and have an estimated
surface area of 0.12 mm
2
and power consumption of
81 mW (Briere et al., 2015). It was shown that
WiNoCoD interconnect has a 0.2-0.3 dB/mm
attenuation with distance over 20-40 GHz band.
These results are derived in the scope of WiNoCoD
project (Briere et al., 2015; Hamieh at al., 2014).
3 INFORMATION THEORETIC
ANALYSIS OF THE PROPOSED
RF INTERCONNECT
3.1 RF Interconnect Capacity
Derivation
In this section, we introduce a brief analysis of
achievable communication capacities between
tilesets and the associated minimum transmission
powers based on the information theory. Information
theory, which is founded by C. Shannon’s seminal
paper (Shannon, 2001) provides the bound for
maximum achievable transmission rate on a
communication channel with the given signal power,
where the probability of error approaches the zero.
This theoretical bound is independent of the utilized
signal protection or correction mechanisms and may
provide a good insight for designers for dimensioning
a reliable communication on a channel. The
information theoretic capacity of a channel can be
written in bits/sec as:
where B is bandwidth in Hz and SNR is Signal-to-
Noise Power Ratio in linear. The power of the noise
P
N
, depends on the temperature and bandwidth. SNR
can be written as the ratio of the received signal power
to the noise power as P
R
=P
N
. P
N
is the Additive White
Gaussian Noise (AWGN) power in the bandwidth.
The AWGN power spectral density in standard room
temperature is -174 dBm/Hz, which we also accept
this value in our calculations (Shankar, 2002).
As we have a immobile and minuscule
environment in contrast with general wireless
communications, we can assume that the only loss on
transmitted signal power is due to distance between
tilesets. As the frequency response is relatively
nonfluctuating, we can assume a single value for
attenuation per distance over all bandwidth. For our
calculations we assume a 0.25 dB/mm attenuation on
the transmission line, which is the average of minimal
and maximal values of 0.2 and 0.3 dB/mm. Hence, the
received signal power can be written as the ratio of
the transmitted signal power to the attenuation due to
the distances between tileset-i and tileset-j:
P
R
= P
T
/ A(d
ij
).
d
ij
being distance in mm between tileset-i and tileset-
j, the resulting attenuation in dB becomes 0.25d
ij
.
Converting this expression in scalar, we can rewrite
the received signal power as a function of distance:
Capacity Analysis of Radio Frequency Interconnect for
Manycore Processor Chips