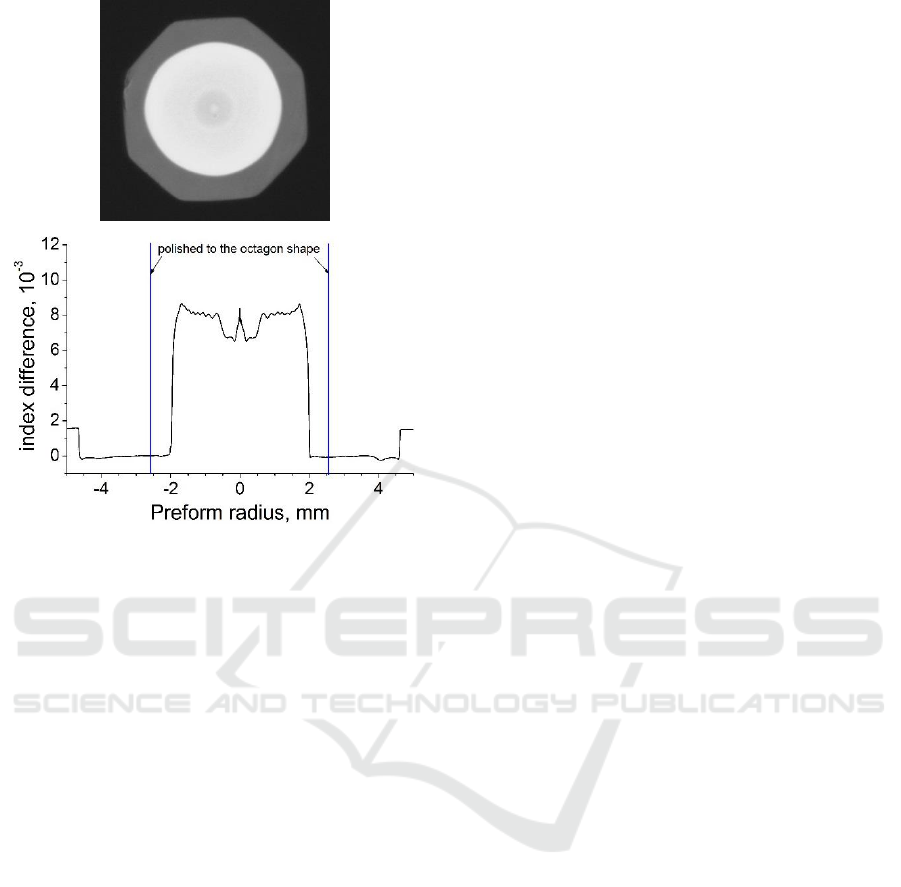
Figure 2: a - Microscope image of the fiber facet; b -
Refractive index profile of the fabricated Er-doped preform.
2.2 Multi-mode Er-doped Fiber
Amplifier at 1565 nm
At first the developed multi-mode Er-doped fiber was
tested in a simple amplifier scheme, shown in Fig.3a.
The standard 2+1-to-1 pump and signal combiner was
used to deliver signal and pump into the Er-doped
fiber. Signal fiber was standard single-mode 8/125
µm double clad fiber. The pump ports were based on
standard 105/125 µm fiber. The pump module has
maximum pump power of 50 W and it wavelength
was stabilized at 976±0.5 nm by an internal volume
Bragg grating. The pump combiner output was
spliced to the developed multi-mode Er-doped fiber.
The multi-mode fiber output was spliced to a standard
multi-mode 105/125 µm fiber. A pump stripper
similar to the described in (Aleshkina S., Kochergina
T.A., 2016) was built at the splice point.
Our calculations has shown that the developed
amplifier has the maximum efficiency when operates
near 1565 nm. An Er-Yb laser with maximum output
power of 0.5 W (limited by available isolator) was
used as a seed source. The length of multi-mode Er-
doped fiber was about 16.7 m that is close to the
optimal one (according to our calculations).
Dependence of output power at 1565 nm on pump
power at 976nm is shown in Fig.3b. The dependence
of output power on input signal (see Fig.3c) shows
that the multi-mode fiber operated in saturation
regime. Both dependences are fairly close to those,
calculated using model and data (i.e. clustering level)
from (Kotov L.V., 2013. Opt. Lett.) and actual
parameters of the developed multi-mode fiber
(core/clad diameters, Er-concentration and grey loss).
The slope pump-to signal conversion efficiency was
found to be as high as 42.4%, which is the highest
ever reported value for high-power Er-doped fiber
lasers pumped at 980 nm. It is still below the
predicted maximum PCE (see Fig.1), which is caused
by increase of grey loss from 5 to 35 dB/km. We
suggest that improvement in preform production
process would result in further increase for the PCE
in the developed multi-mode fiber amplifier.
2.3 Multi-mode Pump Laser at 1535
nm
In addition to a high efficiency, there are other
important demands for the pump source:
compactness, high long-term stability, small size and
low cost. The amplifier scheme presented in previous
paragraph is quite efficient, but it requires operation
of additional seed laser. Presence of the seed laser that
is powerful enough to saturate the amplifier increases
the cost and footprint of the system and makes it more
cumbersome. Moreover, even in saturated regime the
back-reflected signal can strongly affect the amplifier
– a significant signal power might propagate in the
backward direction in this case. Thus, it is preferable
to keep power of the seed laser to be on the level of
4-10% of the output power to ensure a safe operation
regime. When output power would grows to the level
of 100 and even 200 W (see discussion section) the
required seed laser power would exceed limit that is
possible to achieve with Er-Yb lasers. By this reason
in this section we propose a new laser design, free
from aforementioned drawbacks.
The simplest and, therefore, the cheapest scheme
for a fiber laser consists of a gain fiber spliced
between two fiber Bragg gratings (FBGs). However,
it is known that different modes have different
reflection spectra from FBG written in multimode
fiber. As a result, the spectrum of such a multimode
laser has several peaks (Kurkov A.S., 2007), leading
to effective spectral broadening. In addition, this
effect could result in unstable operation because of
mode competition. To ensure a narrow spectrum of a
multimode laser, a master oscillator power amplifier
(MOPA) scheme could be used.
In this work, we propose the new, simple
multimode laser scheme shown in Fig. 4 a. A pump at
High Brightness Multi-Mode Fiber Lasers - A Novel Sources for in-Band Cladding Pumping of Singlemode Fiber Lasers
101