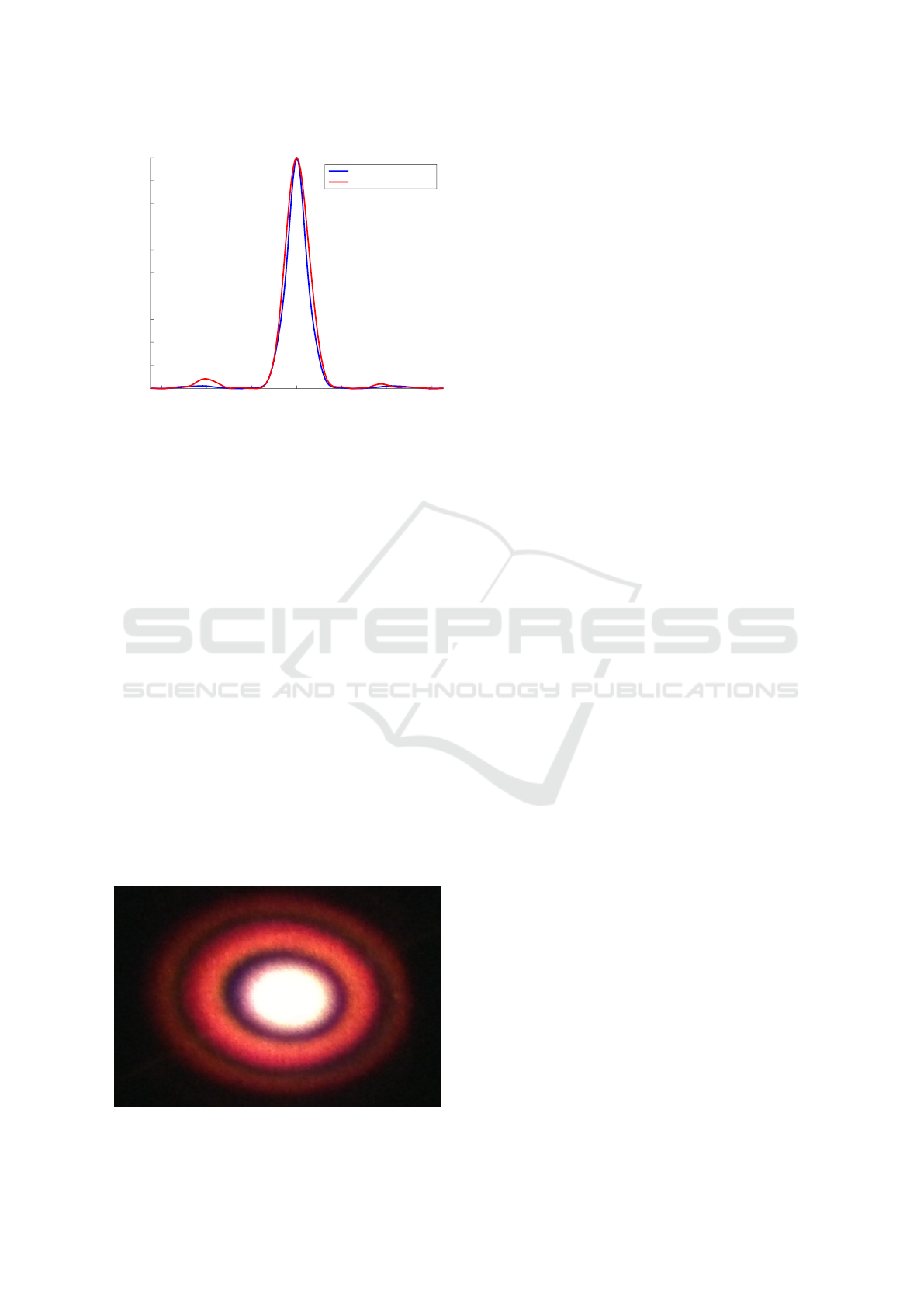
-60 -40 -20 0 20 40 60
Time (fs)
0
0.1
0.2
0.3
0.4
0.5
0.6
0.7
0.8
0.9
1
Norm. intensity
TL: 9.8 fs
Measured: 12.5 fs
Figure 4: Retrieved pulse and transform-limited pulse.
We see that the retrieved pulse has twice the dura-
tion compared with the transform-limited (TL) pulse
(ie., a pulse with same spectrum but with a flat spec-
tral phase).
The pulses generated by MPC are longer than the
pulses generated in a hollow-core fiber (Silva et al.,
2014) by a factor of 3, but nonetheless this kind
of setup has its own advantages, such as compact-
ness (it occupies a small space), simplicity (can be
implemented with off-the-shelf optical components),
robustness (less dependent on alignment, whereas
hollow-core fibers setups normally require feedback
loops to keep a good alignment for a long period of
time), high efficiency, and does not involve the han-
dling of gases within a vaccuum system.
The output power of the MPC process is roughly
50 % of the 140 mW of input average power. The
output beam profile is shown in Figure 5; it is homo-
geneous and most of the power is concentrated in the
central part. Hence, the central part was spatially fil-
tered by an iris (not shown in Figure 1) before being
directed to the measurement setup (d-scan).
Figure 5: Spatial mode.
4 CONCLUSIONS
We successfully compressed and measured ultrashort
laser pulses using the multiplate and dispersion-scan
techniques. We were able to generate a broad spec-
trum capable of supporting transform-limited pulses
down to 9.8 fs from a high peak power laser, which
suggests that the output pulse can be further shortened
if additional phase control or pulse shaping is used.
The spatial mode is homogeneous and stable, and the
MPC process has a good efficiency compared to tra-
ditional hollow-fiber compression setups. The com-
pactness and simplicity of the setup makes the MPC
a good candidate/alternative for application in high-
harmonic generation (HHG), as was demonstrated re-
cently (Huang et al., 2016).
ACKNOWLEDGEMENTS
We acknowledge the funding through the Portuguese
funding agency, Fundac¸
˜
ao para a Ci
ˆ
encia e a Tecnolo-
gia (FCT) through project NORTE-07-0124-FEDER-
000070 (Task 3). The authors thank Francisco Silva
for the d-scan acquisition and retrieval software.
REFERENCES
Alfano, R. (2013). The Supercontinuum Laser Source.
Springer New York.
Anderson, M. E., Monmayrant, A., Gorza, S.-P., Wasyl-
czyk, P., and Walmsley, I. A. (2008). SPIDER: A
decade of measuring ultrashort pulses. Laser Physics
Letters, 5(4):259–266.
B
¨
ohle, F., Kretschmar, M., Jullien, A., Kovacs, M., Mi-
randa, M., Romero, R., Crespo, H., Morgner, U.,
Simon, P., Lopez-Martens, R., et al. (2014). Com-
pression of cep-stable multi-mj laser pulses down to
4 fs in long hollow fibers. Laser Physics Letters,
11(9):095401.
Cheng, Y.-C., Lu, C.-H., Lin, Y.-Y., and Kung, A. H. (2016).
Supercontinuum generation in a multi-plate medium.
Optics Express, 24(7):7224.
Dudley J.M., T. J. e. (2010). Supercontinuum Generation in
Optical Fibers. CUP.
Huang, P.-C., Lu, C.-H., Chen, B.-H., Yang, S.-D., Chen,
M.-C., and Kung, A. H. (2016). EUV continuum
from compressed multiple thin plate supercontinuum.
In Conference on Lasers and Electro-Optics, page
FTu3N.7, Washington, D.C. OSA.
Humbert, G., Wadsworth, W., Leon-Saval, S., Knight, J.,
Birks, T., Russell, P. S. J., Lederer, M., Kopf, D.,
Wiesauer, K., Breuer, E., et al. (2006). Supercontin-
uum generation system for optical coherence tomog-
raphy based on tapered photonic crystal fibre. Optics
express, 14(4):1596–1603.
Dispersion-scan Measurements of the Multiplate Continuum Process
271