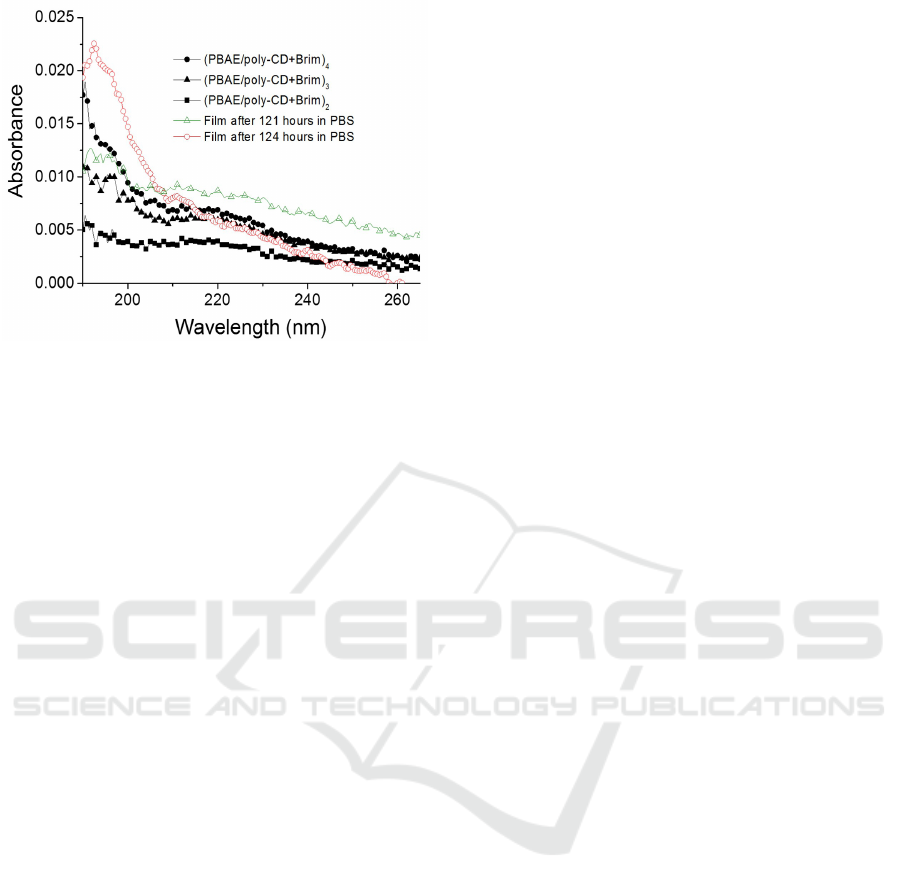
Figure 10: Absorption spectra of a
film with all layers: (PBAE/polyCD +
Brim)
4
/(GO
+
/GO
−
)/(PBAE/polyCD + Brim)
4
and
the spectrum obtained after more than 5 days immersion
time.
4 CONCLUSIONS
A new drug delivery system based on multilayer films
fabricated by the layer-by-layer technique was pre-
sented. This versatile method allowed the fabrication
of a time controlled system introducing in the com-
position of the film an hydrosoluble polymer - poly
(β-amino ester) - and charged graphene oxyde layers.
Both materials are able to control the release of the
studied drug (brimonidine) encapsulated in a poly β-
cyclodextrin but the presence of graphene oxide can
delay the brimonidine release up to 5 days. This is
an important result for the study of time-controlled
drug delivery systems since it allows adaptation of the
number of layers and the film architecture in order to
delay the film desorption, stopping the release of bri-
monidine in the eye, in the way that only the needed
dose will be administrated.
This work developed the first DD LbL films
for glaucoma treatment using biocompatible and
biodegradable materials for the release of precise
amounts of an anti-IOP drug, at determined periods
of time. The high non-compliance level in glau-
coma treatment leads to thousands of individuals to go
blind every year. However, the latest developments on
drug delivery of drugs, with the most varied carriers
have revolutionized the ophthalmic treatments offer-
ing new, improved systems that can control the glau-
coma condition but also substitute the current treat-
ments with daily eye drop application.
ACKNOWLEDGEMENTS
The authors thank Fundac¸ao para a Ci
ˆ
encia e
Tecnologia-Portugal for financial support under the
project UID/EEA/50008/2013 and Post-Doc grant
SFRH/BPD/75338/2010.
REFERENCES
Bosch-Navarro, C., Coronado, E., Mart
´
ı-Gastaldo, C.,
S
´
anchez-Royo, J. F., and G
´
omez, M. G. (2012). Influ-
ence of the ph on the synthesis of reduced graphene
oxide under hydrothermal conditions. Nanoscale,
4(13):3977.
Choi, W., Choi, J., Bang, J., and Lee, J. H. (2013). Layer-
by-layer assembly of graphene oxide nanosheets on
polyamide membranes for durable reverse-osmosis
applications. ACS Applied Materials and Interfaces,
5(23):12510–12519.
Decher, G., Hong, J., and Schmitt, J. (1992). Buildup of
ultrathin multilayer films by a self-assembly process:
Iii. consecutively alternating adsorption of anionic and
cationic polyelectrolytes on charged surfaces. Thin
Solid Films, 210-211:831–835.
Deng, X., Zheng, N., Song, Z., Yin, L., and Cheng, J.
(2014). Trigger-responsive, fast-degradable poly(β-
amino ester)s for enhanced dna unpackaging and re-
duced toxicity. Biomaterials, 35(18):5006–5015.
Enscore, D., Hopfenberg, H., Stannett, V., and Berens, A.
(1977). Effect of prior sample history on n-hexane
sorption in glassy polystyrene microspheres. Polymer,
18(11):1105–1110.
European Glaucoma Society (2014). Terminolology and
guidelines for glaucoma. European Glaucoma Soci-
ety, 4th edition edition.
Ferreira, Q., Braganca, A. M., Alcacer, L., and Morgado, J.
(2014). Conductance of well-defined porphyrin self-
assembled molecular wires up to 14 nm in length.
Journal of Physical Chemistry C, 118(13):7229–7234.
Ferreira, Q., Gomes, P., Maneira, M., Ribeiro, P., and Ra-
poso, M. (2007). Mechanisms of adsorption of an azo-
polyelectrolyte onto layer-by-layer films. Sensors and
Actuators B: Chemical, 126(1):311–317.
Ferreira, Q., Ribeiro, P. A., Oliveira, O. N., and Raposo, M.
(2012). Long-term stability at high temperatures for
birefringence in pazo/pah layer-by-layer films. ACS
Applied Materials & Interfaces, 4(3):1470–1477.
Fick, A. (1995). On liquid diffusion. Journal of Membrane
Science, 100(1):33–38.
Hal
´
asz, K., Hosakun, Y., and Cs
´
oka, L. (2015). Reduc-
ing water vapor permeability of poly(lactic acid) film
and bottle through layer-by-layer deposition of green-
processed cellulose nanocrystals and chitosan. Inter-
national Journal of Polymer Science, 2015:1–6.
Holowka, E. P. and Bhatia, S. K. (2014). Drug Delivery,
volume 197 of Handbook of Experimental Pharma-
cology. Springer New York.