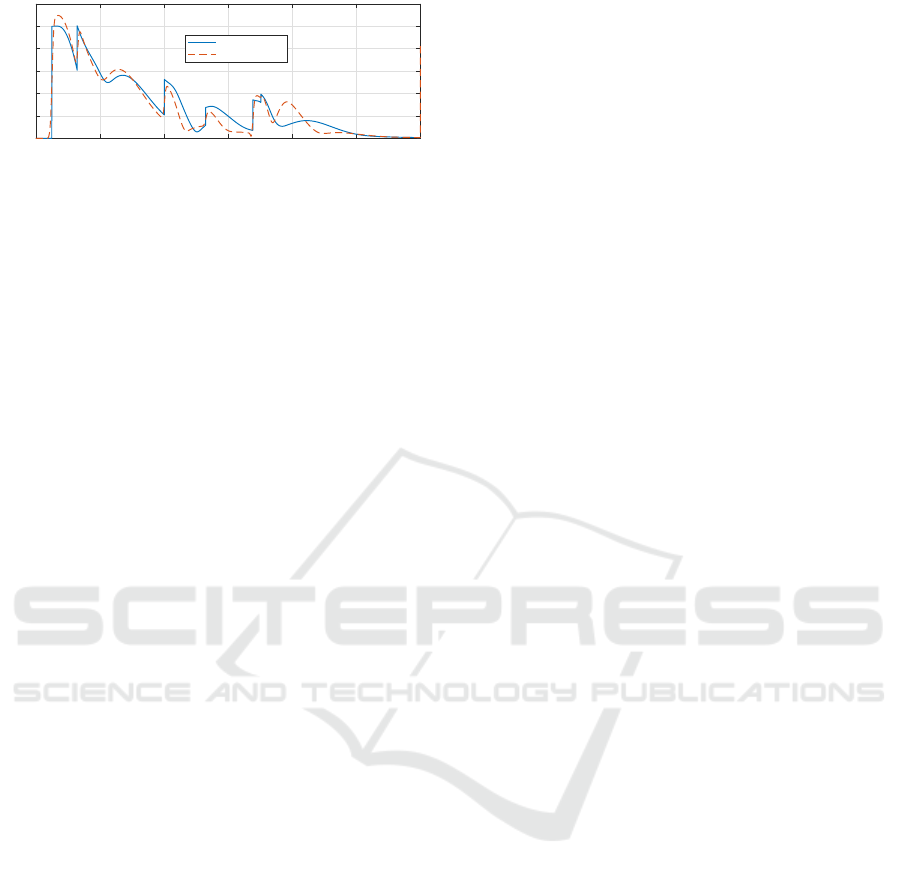
0 2 4 6 8 10 12
t (s)
0
0.2
0.4
0.6
0.8
1
1.2
Absolute position error (m)
P1 absolute error
P2 absolute error
Figure 11: Absolute position error of the quadcopter during
the VTOL test.
7 CONCLUSIONS
The approach proposed in this work shows how to im-
prove a classic control system dedicated to VTOL op-
erations over a moving target. In particular its shown
that the trajectory tracking error might be improved
and the UAS is able to perform a smooth VTOL ma-
noeuvre over the USV.
The stability and softness provided by the dy-
namic movement primitives might be able to improve
navigation manoeuvres subject to waves or even with
wind gusts, and including dynamic obstacle avoid-
ance capabilities. In spite of the early characteristic
of this experimentation, the preliminary results hint
of a sizeable improvement once more characteristics
of the DMP are introduced into the control architec-
ture.
Better performance could be expected, especially
when performing repetitive cyclic and rhythmical
tasks, typical in UAS based sensing techniques.
ACKNOWLEDGMENTS
The research leading to these results has received
funding from the RoboCity2030-III-CM project
(Rob
´
otica aplicada a la mejora de la calidad de vida
de los ciudadanos. fase III; S2013/MIT-2748), funded
by Programas de Actividades I+D en la Comunidad
de Madrid and cofunded by Structural Funds of the
EU.
REFERENCES
Davidson, K. and Schiff, L. (1946). Turning and course
keeping qualities of ships. Transactions of SNAME,
4:49.
Djapic, V., Prijic, C., and Bogartz, F. (2015). Autonomous
takeoff landing of small uas from the usv. In OCEANS
2015 - MTS/IEEE Washington, pages 1–8.
Erginer, B. and Altug, E. (2007). Modeling and pd control
of a quadrotor vtol vehicle. In 2007 IEEE Intelligent
Vehicles Symposium, pages 894–899.
Fossen, T. I. (2011). Handbook of marine craft hydrody-
namics and motion control. John Wiley & Sons.
Gams, A., Ijspeert, A. J., Schaal, S., and Lenar
ˇ
ci
ˇ
c, J.
(2009). On-line learning and modulation of periodic
movements with nonlinear dynamical systems. Au-
tonomous robots, 27(1):3–23.
Heriss
´
e, B., Hamel, T., Mahony, R., and Russotto, F.-X.
(2012). Landing a vtol unmanned aerial vehicle on a
moving platform using optical flow. IEEE Transac-
tions on Robotics, 28(1):77–89.
Ijspeert, A. J., Nakanishi, J., Hoffmann, H., Pastor, P., and
Schaal, S. (2013). Dynamical movement primitives:
learning attractor models for motor behaviors. Neural
computation, 25(2):328–373.
Ijspeert, A. J., Nakanishi, J., and Schaal, S. (2003). Learn-
ing attractor landscapes for learning motor primitives.
Advances in neural information processing systems,
pages 1547–1554.
Nemec, B., Tamo
ˇ
si
¯
unait
˙
e, M., W
¨
org
¨
otter, F., and Ude, A.
(2009). Task adaptation through exploration and ac-
tion sequencing. In Humanoid Robots, 2009. Hu-
manoids 2009. 9th IEEE-RAS International Confer-
ence on, pages 610–616. IEEE.
Omar, V. A. (2017). Modelling and control of a uav-usv
collaboration scheme for fluvial operations. Bache-
lor’s thesis, Univ. Carlos III de Madrid.
Ore, J.-P., Elbaum, S., Burgin, A., Zhao, B., and Detweiler,
C. (2015). Autonomous Aerial Water Sampling, pages
137–151. Springer International Publishing, Cham.
Perez, T. and Blanke, M. (2002). Mathematical ship mod-
elling for control applications. Ørsted-DTU, Automa-
tion.
Pinto, E., Santana, P., Marques, F., Mendonc¸a, R.,
Lourenc¸o, A., and Barata, J. (2014). On the design
of a robotic system composed of an unmanned surface
vehicle and a piggybacked vtol. In Doctoral Confer-
ence on Computing, Electrical and Industrial Systems,
pages 193–200. Springer.
Schaal, S., Peters, J., Nakanishi, J., and Ijspeert, A. (2005).
Learning movement primitives. In Robotics Research.
The Eleventh International Symposium, pages 561–
572. Springer.
Weaver, J. N., Frank, D. Z., Schwartz, E. M., and Arroyo,
A. A. Uav performing autonomous landing on usv
utilizing the robot operating system.
ICINCO 2017 - 14th International Conference on Informatics in Control, Automation and Robotics
396