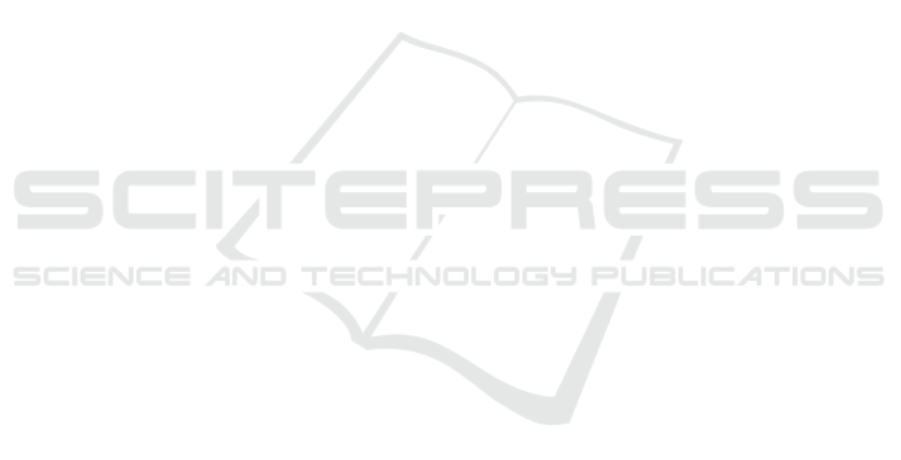
the lifelong medical follow up of patients it becomes
clear that, whilst primary medical care provides vi-
tal treatment, patients need for inclusion is likely to
benefit strongly also from the collaboration between
medical expertise, neuroscience and advances in neu-
rotechnology.
Technical adaptations were performed in a commer-
cially available EEG system to conform to the follow-
ing requirements:
• unobtrusive visual appearance that is not imme-
diately associated with a clinical examination de-
vice.
• short preparation time: < 5 minutes.
• comfortable wearing for up to 30 minutes.
• easily adapted to different head sizes (from chil-
dren to adults).
• electrodes positioning according to the 10-20 sys-
tem (Homan et al., 1987) and covering the major-
ity of sensorimotor areas.
• resistance to hygienic treatment.
• positive reception/good acceptance of the device
by the participants.
2.2 Design Concept and Realization
Standard EEG recording equipment for scientific and
medical purposes usually consist of a cap of flexi-
ble fabric with electrode placeholders, an amplifier
connected to the electrodes via cables, and a com-
puter connected to the amplifier recording and stor-
ing the data (e.g. BrainProducts actiChamp). The cap
setup allows for flexible and precise positioning of the
electrodes and is comfortable to wear. However, de-
spite the high data precision achieved with these sys-
tems, some technical aspects make them non-optimal
for recordings in some clinical populations. Most
such systems use gel-based electrodes which require
long setup times, exceeding the patient’s endurance,
and are as such not usable. Alternative systems use
dry electrodes (e.g. Guger Technologies g.Nautilus)
which significantly reduce preparation time. How-
ever, dry electrodes require firm contact pressure of
the electrode on the scalp to yield good conductiv-
ity and as such significantly reduce wearing com-
fort. Moreover, dry electrodes are more susceptible to
noise and measurement artifacts than gel-based elec-
trodes. At last, the chin strap for closing and tight-
ening the cap can cause feelings of suffocation and
might not be tolerated by some patients. Also, the
chin-strap makes signal acquisition prone to artifacts
resulting from head and face movements as well as
talking. Patients with CP, especially adolescents, are
unlikely to sit still for long time. Some systems do not
make use of a cap setup, but rather a frame or flexi-
ble headband design, such as the Quasar HMS, or the
emotiv EPOC. These systems however were also in-
adequate for the envisaged group, partially because of
their obtrusive appearance, partially because of lim-
ited flexibility for electrode positioning. None of the
commercially available systems fullfilled all require-
ments which motivated the need for a costumized de-
sign.
As a compromise between flexible cap and stiff
frame setup which does not require a chin strap we
decided for a design mimicking headphones (see Fig-
ure 1). Headphones have natural unobtrusive appear-
ance as people are familiar with using them for mu-
sic listening. Furthermore, headphones apply contact
pressure around the ears which has proven comfort-
able to wear and allow for tight sit. In addition, the
headphones allow the recording electrodes to be em-
bedded within their headband and consequently to be
naturally positioned over motor areas, necessary to as-
sess sensorimotor-related brain activity.
As for sensors and electronics we decided to re-
use a worn-off emotiv EPOC device. Despite the orig-
inal purpose of the emotiv EPOC for gaming and en-
tertainment, there have been numerous scientific pa-
pers making use of the system. The system has sys-
tematically been validated with regard to the use for
scientific purposes, e.g. in (Hairston et al., 2014) and
(Debener et al., 2012). This shows that despite lower
signal quality, the system delivers usable data in a
wide spectrum of applications. The emotiv EPOC
sensor technology makes use of a semi-dry solution,
namely felt pads soaked with saline solution estab-
lishing conductivity between the proband’s scalp and
the gold-coated electrodes. The electrodes require a
certain contact pressure but the soft felt pads allow
for comfortable wearing. The emotiv EPOC headset
is a one-size for all frame with a fixed positioning of
the electrodes. Its original layout has mainly channels
over the pre-frontal areas.
We freed sensors and electronics from the original
plastic frame and included the raw modules into our
headphones setup. A flexible two-layer sheet made of
washable fabric was mounted in between the left and
right earpiece, which are held together by two curved
threaded metal bars. The two-layer sheet embeds and
hides 13 (of original 14) EEG electrodes and the con-
necting cables to the electronics. One channel was
spared for capturing vertical eye-movement via elec-
trooculogram (EOG) signals (see Figure 1). This al-
lows for either online or post-hoc eye-movement ar-
tifact reduction. The right earpiece was used as the
housing for electronics, leaving enough space to fill