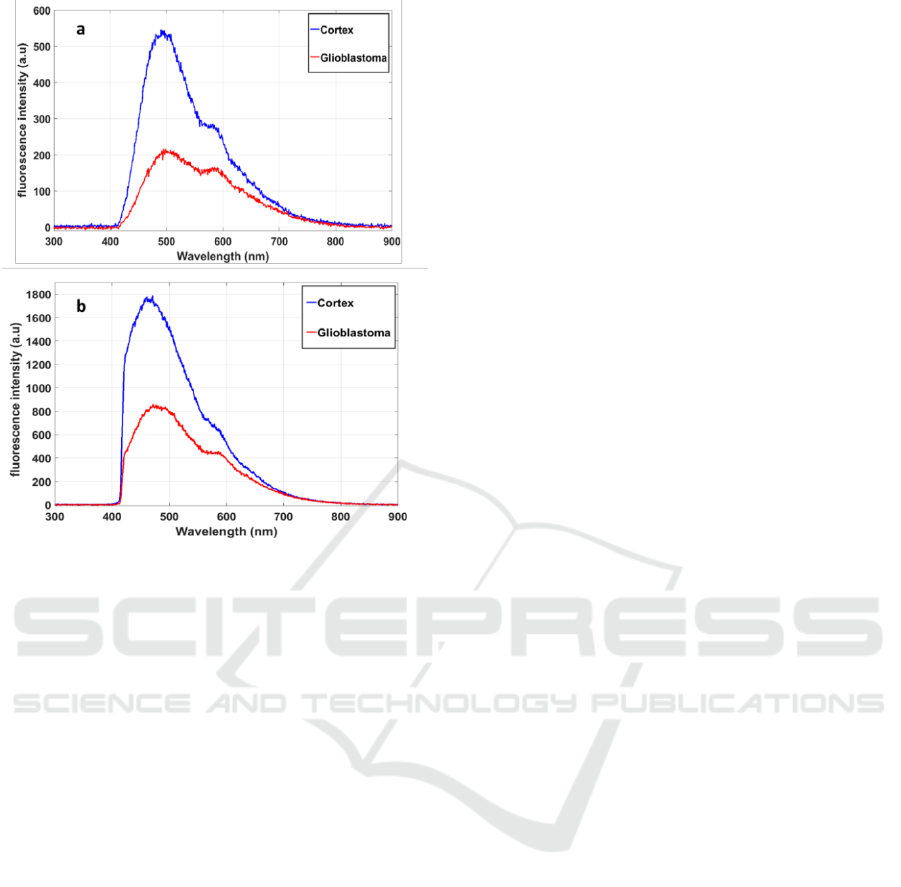
Figure 3: Fluorescence spectra of glioblastoma and cortex
tissues excited with 405 nm (a) and 375nm (b)
wavelength.
5 DISCUSSION
In this preliminary study, we compared fixed cortex
and glioblastoma samples from adult patients using
two types of measurements. First, we observed that
the scattering and absorption coefficient are higher
in glioblastoma samples than in cortex samples. This
could be related to the fact that glioblastoma tissues
have denser vascularization and more collagen fibres
than cortex. This vascularization could be the source
of light scattering in such tissues. Added to the fact
that glioblastoma tissues contain neovascularization
which could affect the absorption coefficient.
By referring to our previous spectral studies on
rats brain tumors and healthy tissues (Haidar et al.,
2015) and on human brain tissues (Zanello et al.,
2017) using 405 nm excitation wavelength, the five
excited fluorophores are more concentrated in the
healthy tissues than in the glioblastoma tissues. For
that, the total fluorescence signal in cortex tissue is
higher than in glioblastoma. The same trend was
shown using 375 nm excitation. At this excitation
wavelength the absorption efficient section of
NADH and the FAD is higher than at 405nm
excitation wavelength. So, we optimise the
efficiency of excitation of this two endogenous
molecules and specially the excitation of NADH.
We can show also that endogenous fluorescence
from healthy tissues is higher than glioblastoma
tissues. This observation is of paramount important
as spectral response can discriminate healthy from
glioblastoma tissues. This is in accordance with the
literature of spectral analysis on freshly resected
tissues (Zanello et al., 2017).
Here, we show bimodal quantitative
measurements on the same tissues. These
measurements prove the power of optical detection,
based on endogenous fluorescence of frozen tissues
to discriminate healthy from tumoral tissues.
This opens a promising door in the detection of
tumors margins. It proved also how important is the
multimodality to detect with reliability the nature
tissues area, highlighting the importance of our
optical endomicroscope.
In the future studies, we will extend our cohort
by examining more types of human brain tumors and
also extend our study to freshly extracted samples.
ACKNOWLEDGEMENTS
This work is supported by a Plan Cancer with
Physicancer program grant “IMOP,” a “Défi
instrumental” program grant from CNRS, the Institut
National de Physique Nucléaire et de Physique des
Particules (IN2P3), and the “Ligue contre le cancer”.
REFERENCES
Bevilacqua, F., Piguet, D., Marquet, P., Gross, J.D.,
Tromberg, B.J., Depeursinge, C., 1999. In vivo local
determination of tissue optical properties:
applications to human brain. Appl. Opt. 38, 4939–
4950.
Haidar, D.A., Leh, B., Zanello, M., Siebert, R., 2015.
Spectral and lifetime domain measurements of rat
brain tumors. Biomed. Opt. Express 6, 1219–1233.
Ibrahim, A., Poulon, F., Habert, R., Lefort, C., Kudlinski,
A., Haidar, D.A., 2016a. Characterization of fiber
ultrashort pulse delivery for nonlinear
endomicroscopy. Opt. Express 24, 12515–12523.
Ibrahim, A., Poulon, F., Melouki, F., Zanello, M., Varlet,
P., Habert, R., Devaux, B., Kudlinski, A., Haidar,
D.A., 2016b. Spectral and fluorescence lifetime
endoscopic system using a double-clad photonic
crystal fiber. Opt. Lett. 41, 5214–5217.
Poulon, F., Mehidine, H., Juchaux, M., Varlet, P., Devaux,
B., Pallud, J., Abi Haidar, D., 2017. Optical
properties, spectral, and lifetime measurements of
Identifying Isolated Glioblastoma Tissues in Human Patients through Their Optical and Spectral Properties
139