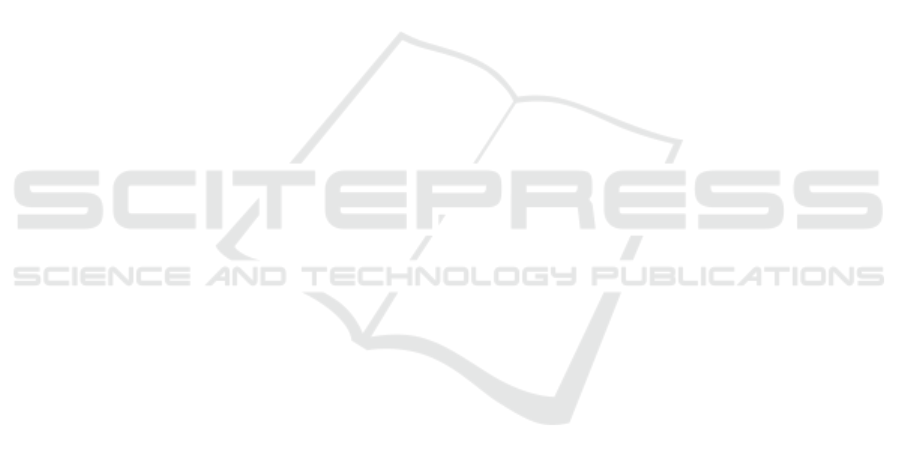
medium-sized trams – have become an attractive
proposal of urban public transport in cities like
Medellin in Colombia, and Caracas, in Venezuela,
due to the fact that they provide adequate transport
over mountainous land, rivers, historic and densely
residential areas (Bergerhoff and Perschon, 2013).
When it comes to road public transport, bus rapid
transit (BRT) has been implemented as a new
transport system in cities where the other options are
not available. BRT, which was born in Curitiba, has
set an example for cities like Quito, Bogotá, Pereira,
Sao Paulo, Santiago de Chile, and Guayaquil,
showing to be an efficient and sustainable solution
in congested cities (Jirón, 2013).
The solution to transport problems, and selection
of one or more options – of the nine afore mentioned
– can be analyzed through trips or transport models.
These models are tools that provide a systematic
referential framework to represent how trip demand
changes in response to different presumptions, and
allow evaluation of advantages and disadvantages of
different transport alternatives (Castiglione, et al.,
2015).
Transport modeling includes instruments,
strategies, and solutions that have an influence on
the results of vehicle congestion, times and trip
speeds, which makes it difficult to determine which
the best option is; just focusing on one criterion
limits taking advantage of all these instruments.
Conversely, evaluation of transport model variables,
using multi-criteria decision analysis (MCDA) helps
to find the best option that suit the goal (Nosal and
Solecka, 2014). One of the most known MCDA is
analytic hierarchy process (AHP).
The number of researches using AHP has
increased over the last decade, especially in
mathematical methods, computer science and
management studies. Furthermore, AHP has been
successfully applied in the management of limited
resources, the transportation sector, strategic
planning and in the area of logistics (Emrouznejad
and Marra, 2017). In optimization of the public
transport net, multifactor analysis through AHP
adjusts better since more reasonable and practical
results are attained (Xiaowei, et al., 2010).
The future view is the search of sustainable
transport, which is essential to achieve most – if not
all – of the goals set by the United Nations
Organization for Sustainable Development.
However, climate change debate concentrates on
energy and industrial activity, setting aside transport,
without considering that the latter is responsible of a
fourth of greenhouse gas emissions per year,
globally (United Nations, 2015). In Latin America,
the amount of CO
2
emissions is around 357
thousand tons per day, being individual transport
vehicles, the ones producing higher amounts than
public transport vehicles (CAF, 2016).
This study proposes selecting a sustainable
transport methodology for an Ecuadorian city,
considering the variables travel time, waiting time,
pollutants emission and noise, technology, system
costs, among others; part of the search for
sustainable transport as an applied case in the city of
Ambato.
2 METHODOLOGY
Conventional multi-criteria decision making
considers both quantitative and qualitative criteria;
there exist methods for the quantitative approaches
and other for the qualitative ones. However, the
problem of this study, about selecting a sustainable
transport methodology, combines quantitative and
qualitative criteria for valuation of the best option.
For this reason, AHP method is used, which allows
handling both approaches. This method, developed
by Thomas L. Saaty in 1980, makes paired
comparisons at a scale implemented by himself. In
the AHP solution, the problem is modeled with a
hierarchy; first, the object is defined, becoming the
highest. Then, the criteria and sub criteria are
established, locating these in the intermediate level.
Afterwards, the alternatives are identified in the
lower level, to finally establish the priorities of each
alternative and choose the best option. (Saaty, 1980).
After the alternatives are compared with each
other in terms of each one of the decision criteria,
the method evaluates each alternative with respect to
each criterion and then multiples that evaluation by
the importance of the criterion. This product is
summed over all the criteria for the particular
alternative to generate the rank of the alternative.
Mathematically:
Where R
i
is the rank of the i
th
alternative, a
ij
is
the actual value of the i
th
alternative in terms of the
j
th
criterion, and w
j
is the weight or importance of the
j
th
criterion (Nguyen, 2014).
The proposed objective in the AHP model of this
research is the selection of the best model of
sustainable urban public transport, based on results
and experiences of other cities.
Impacts Analysis towards a Sustainable Urban Public Transport System
39