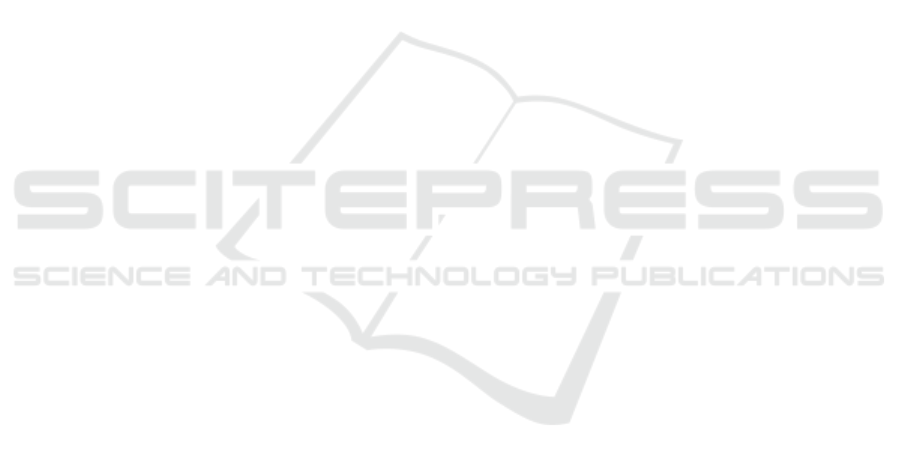
Miniaturized Surface Plasmon Resonance based Sensor System
Peter Hausler
1
, Christa Genslein
2
, Carina Roth
1
, Thomas Vitzthumecker
1
, Thomas Hirsch
2
and Rudolf Bierl
1
1
Sensorik-ApplikationsZentrum,Ostbayerische Technische Hochschule Regensburg,
Franz-Mayer-Str. 1, Regensburg, Germany
2
Institute of Analytical Chemistry, Chemo- and Biosensors, University of Regensburg,
Universitätsstr. 31, Regensburg, Germany
Keywords: Surface Plasmon Resonance Spectroscopy, Sensor, SPR-Imaging, Miniaturization, Micro-Opto-Electro-
Mechanical Systems.
Abstract: We describe the miniaturization of the Surface Plasmon Resonance (SPR) technology which mainly finds its
applications in pharmaceutical screening and biotechnology so far. SPR spectroscopy is a label-free, non-
destructive and highly sensitive measurement principle detecting changes in the refractive index in striking
distance to a gold surface. A transfer of this technology to a miniaturized sensor will broaden the range of
possible applications. A promising feature which is included in the miniaturized system is the angle-dependent
recording of the SPR signals without moving parts. Commercial SPR assays are mainly working with a small
number of sensing spots. In contrast, the SPR imaging system shown here will allow to use an array of many
sensing spots. In combination with chemical receptors designed as an artificial nose, the simultaneous
detection of many analytes is envisioned for future applications.
1 INTRODUCTION
Surface Plasmon Resonance (SPR) technology is
label free, non-destructive and highly sensitive
(Schasfoort, 2017). Due to these properties, SPR is an
attractive measurement principle for chemical
sensors (Homola, 2003). Nevertheless, there are some
drawbacks limiting its applications so far: Classical
measurement setups are mainly designed for being
used in laboratories and therefore they are very
expensive. The high temperature sensitivity and the
need of trained personal for its operation impede
reliable in-field sensing. Motivated by this we
developed a miniaturized sensor system, which is
able to operate automated in a wide range of
temperature.
The system is based on an imaging read-out
principle enabling a large number of sensor spots on
a small area for addressed parallel online detection.
The individual sensing spot can be designed and
chemically functionalized as an artificial nose
enabling the identification of several analytes in a
complex matrix without labelling. In classical SPR
setups a laser is used as light source, which is replaced
by a modified light emitting diode. While using a
semiconductor laser to illuminate a surface one will
get speckles. If it comes to very small sensing spots,
speckles will ruin your sensing signal. Light,
generated by a LED does not exhibit speckles due to
the missing coherence.
2 PRINCIPLE
While a thin gold film is irradiated by light, typically
the entire light will be reflected (Figure 1). However,
if the light is p-polarised and the angle of incidence is
altered, one can see a narrow dip in the intensity of
the reflected light. This dip is indicating that at this
certain angle of incidence (SPR angle) surface
plasmons are excited. The SPR angle mainly depends
on the refractive index in close proximity to the gold
film which is deposited on coupler, usually a glass
prism. Therefore, the refractive index on one side of
the gold is constant, which means that any variations
in the chemical composition – and therefore in the
refractive index – next to the other side of the gold
film is determining the position of the SPR-angle.
Selectivity to a special molecule of interest is
generated by a chemical functionalization of the gold
Hausler, P., Genslein, C., Roth, C., Vitzthumecker, T., Hirsch, T. and Bierl, R.
Miniaturized Surface Plasmon Resonance based Sensor System.
DOI: 10.5220/0006555400630066
In Proceedings of the 6th International Conference on Photonics, Optics and Laser Technology (PHOTOPTICS 2018), pages 63-66
ISBN: 978-989-758-286-8
Copyright © 2018 by SCITEPRESS – Science and Technology Publications, Lda. All rights reserved
63