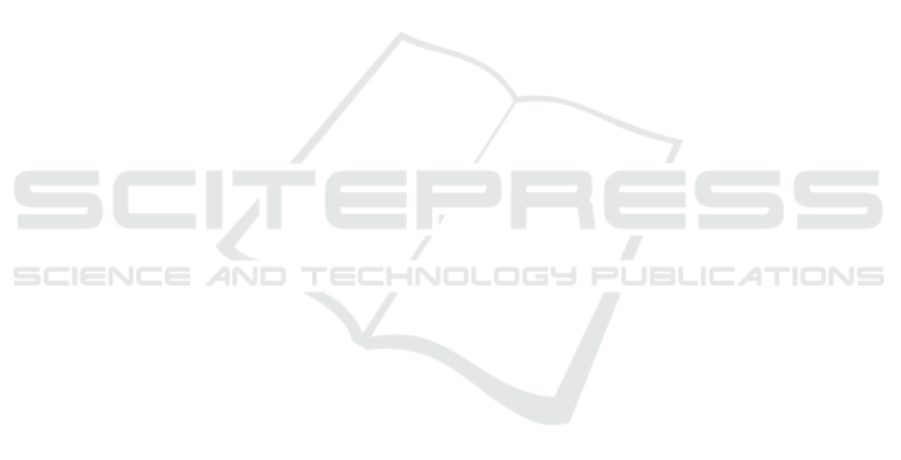
REFERENCES
Abbadie, a., Hartmann, J. M., and Brunier, F. (2007). A
review of different and promising defect etching
techniques: from Si to Ge. The Electrochemical Society
A, 10(1):3–19.
Bal, H. K., Brodzeli, Z., Dragomir, N. M., Collins, S. F.,
and Sidiroglou, F. (2012). Uniformly thinned optical
fibers produced via HF etching with spectral and
microscopic verification. Applied optics, 51(13):2282–
2287.
Baude, E. and Branco, P. (2013). Numerical study of
tapered fiber optics as evanescent field sensors.
SBMO/IEEE MTT-S International Microwave &
Optoelectronics Conference (IMOC), 1–5.
Bosch, M. E., Jes´us, A., S´anchez, R., Rojas, F. S., and
Ojeda, C. B. (2007). Recent development in optical
fiber biosensors. Sensors, 7:797–859.
Ferri, S., Kojima, K., and Sode, K. (2011). Review of
glucose oxidases and glucose dehydrogenases: a bird’s
eye view of glucose sensing enzymes. Diabetes Science
and Technology, 5(5):1068–1076.
Fielding, A. J. and Davis, C. C. (2002). Tapered single-
mode optical fiber evanescent coupling. IEEE
Photonics Technology Letters, 14(1):53–55. G.
Guo, Z. J., Boyter, C., Cohoon, G., Salik, E., and Lin, W.-
J. (2011). Detection of botulinum neurotoxin by tapered
fiber optic biosensor. GSTF Journal of BioSciences
1(1):36–42.
Haddock, H. S., Shankar, P. M., and Mutharasan, R. (2003).
Fabrication of biconical tapered optical fibers using
hydrofluoric acid. Materials Science and Engineering
B: Solid-State Materials for Advanced Technology.
Karapetyan, K. (2012). Single optical modal
interferometer. PhD thesis.
Khan, A. Y., Noronha, S. B., and Bandyopadhyaya, R.
(2014). Glucose oxidase enzyme immobilized porous
silica for improved performance of a glucose biosensor.
Biochemical Engineering Journal, 91:78–85.
Klonoff, D. C. (2012). Overview of fluorescence glucose
sensing: f Technology with a bright future. Journal of
Diabetes Science and Technology, 6(6):1242–1250.
Ko, S., Lee, J., Koo, J., Joo, B. S., Gu, M., and Lee, J. H.
(2016). Chemical wet etching of an optical fiber using
a hydrogen fluoride-free solution for a saturable
absorber based on the evanescent field interaction.
Journal of Lightwave Technology, 34(16):3776–3784.
Leung, A., Shankar, P. M., and Mutharasan, R. (2008a).
Label-free detection of DNA hybridization using gold-
coated tapered fiber optic biosensors (TFOBS) in a flow
cell at 1310 nm and 1550 nm. Elsevier, 131:640–645.
Leung, A., Shankar, P. M., and Mutharasan, R. (2008b).
Model protein detection using antibody-immobilized
tapered fiber optic biosensors (TFOBS) in a flow cell at
1310 nm and 1550 nm. Sensors and Actuators, B:
Chemical, 129(2):716–725.
Liébana S, and Drago G.A. (2016). Bioconjugation and
stabilisation of biomolecules in biosensors. Essays in
Biochemistry, 60(1):59–68.
Kenny, R. P., Birks, T. A, and Oakley, K. P. (1991). Control
of optical fibre taper shape. Electronics Letters, 27.
Polynkin, P., Polynkin, A., Peyghambarian, N., and
Mansuripur, M. (2005). Evanescent field-based optical
fiber sensing device for measuring the refractive index
of liquids in microfluidic channels. Optics Letters,
30(11):1273–1275.
Qiang, Z., Junyang, L., Yanling, Y., Libo, G., and
Chenyang, X. (2014). Micro double tapered optical
fiber sensors based on the evanescent field-effect and
surface modification. Optik, 125(17):4614–4617.
Qiu, H. W., Xu, S. C., Jiang, S. Z., Li, Z., Chen, P. X., Gao,
S. S., Zhang, C., and Feng, D. J. (2015). Applied surface
science a novel graphene-based tapered optical fiber
sensor for glucose detection. Elsevier, 329:390–395.
Ruan, Y., Foo, T. C., Warren-Smith, S., Hoffmann, P.,
Moore, R. C., Ebendorff-Heidepriem, H., and Monro,
T. M. (2008). Antibody immobilization within glass
microstructured fibers: a route to sensitive and selective
biosensors. Optics Express, 16(22):18514–18523.
Salceda-Delgado, G., Monzon-Hernandez, D., Martinez-
Rios, a., Cardenas-Sevilla, G. a., and Villatoro, J.
(2012). Optical microfiber mode interferometer for
temperature-independent refractometric sensing.
Optics Letters, 37(11):1974.
Shi, J., Xiao, S., Yi, L., and Bi, M. (2012). A sensitivity-
enhanced refractive index sensor using a single-mode
thin-core fiber incorporating an abrupt taper. Sensors,
12(4), 4697–4705.
World Health Organization (2016). Diabetes Global
Report. Technical report.
Yadav, T. K., Narayanaswamy, R., Abu Bakar, M. H.,
Kamil, Y. M., and Mahdi, M. A. (2014). Single mode
tapered fiber-optic interferometer based refractive
index sensor and its application to protein sensing.
Optics Express, 22(19):22802.
Zibaii, M. I., Latifi, H., Karami, M., Gholami, M., Hosseini,
S. M., and Ghezelayagh, M. H. (2010). Non-adiabatic
tapered optical fiber sensor for measuring the
interaction between a-amino acids in aqueous
carbohydrate solution. Measurement Science and
Technology, 21(10):105801.
Tapered Fibre Optic Biosensor (TFOBS) by Optically Controlled Etching for Label-Free Glucose Concentration Monitoring - Biomedical
Optics
173