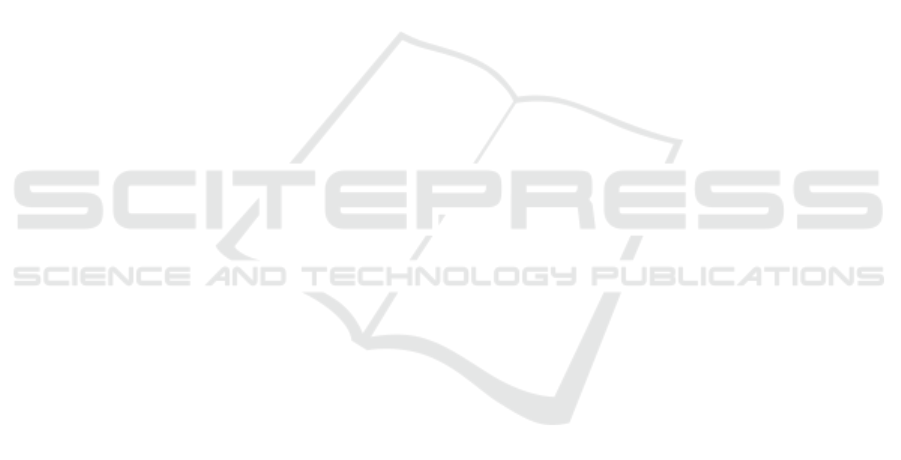
ISO 11539, 1999. Ophthalmic Optics- Contact Lenses-
Classification of Contact Lenses and Contact Lens
Material.
Tranoudis, I., Efron, N., 2004. Water properties of soft
contact lens materials, Contact Lens Anterior Eye, 27,
193-208.
Sweeney, D., 2006, Silicone Hydrogels: The Evolution of a
Revolution, Contact Lens Spectrum.
Jones, L., 2002, Modern Contact Lens Materials: A Clinical
Performance Update, Contact Lens Spectrum.
Cerle J., 1972, Hydrophilic lenses for continuous wearing,
Clinical and Experimental Optometry, 55, 343-346.
Harvitt, D. M., Bonanno, J. A., 1998 Minimum contact lens
transmissibility values for daily and extended wear
contact lenses, Optometry and Vision Science Journal,
75, 189.
Alvord, L., Court, J., Davis, T., Morgan, C. F., Schindhelm,
K., Vogt, J., Winterton, L., 1998, Oxygen Permeability
of a New Type of High Dk Soft Contact Lens Material,
Optometry and Vision Science, 75, 30-36.
Efron, N., Morgan, P. B., Cameron, I. D., Brennan, N. A.,
Goodwin, M., 2007, Oxygen Permeability and Water
Content of Silicone Hygrogel Contact Lens Materials,
Optometry and Vision Science, 84, 328-337.
ISO International Standard 9913-1, 1996, Optics and
optical instruments, Determination of Oxygen
Permeability and Transmissibility by the Fatt Method,
Geneva, Switzerland: International Organization for
Standardization.
ISO International Standard 9913-2, 2000, Optics and
optical instruments, Determination of Oxygen
Permeability and Transmissibility by the Coulometric
Method, Geneva, Switzerland: International
Organization for Standardization.
ISO International Standard 18369-4, 2006, Ophthalmic
optics-Contact lenses. Part 4: Physicochemical
Properties of Contact Lens Materials, Geneva:
International Organization for Standardization.
Lee, S. E., Kim, S. R., Park, M., 2015, Oxygen permeability
of soft contact lenses in different pH, osmolality and
buffering solution, International Journal of
Ophthalmolgy, 8, 1037-1042.
Lewandowska, M., Jasińska-Kwaśnik, W., Jóźwik, A.,
Siedlecki, D., 2015, Measurement of oxygen
permeability of contact lenses based on analysis of
porosity, Interdisciplinary Journal of Engineering
Science, III, 1-5.
Hung, C. W., Huang, K. C., Tsai, H. Y., Lin, Y. H., Shum,
P. J-T, 2017, Development of Instant Measuring Model
for Oxygen Permeability and Water Content of
Hydrogel Contact Lens, Intertional Conference on
Photonics, Optics and Laser Technology, 1, 175-82.
Hale, G. M., Querry, M. R., 1973, Optical constants of
water in the 200nm to 200µm wavelength region,
Applied Optics, 12, 555-563.
Taiz, L., Zieger, E., 2015, Plant Physiology and
Development, Sinauer Associates, Inc.6
th
Edition.
Lira, M., Pereira, C., Real Oliveira, M. E., Castanheira, E.
M., 2014, Importance of contact lens power and
thickness in oxygen transmissibility, Contact Lens &
Anterior Eye, 38, 120-126.
PHOTOPTICS 2018 - 6th International Conference on Photonics, Optics and Laser Technology
212