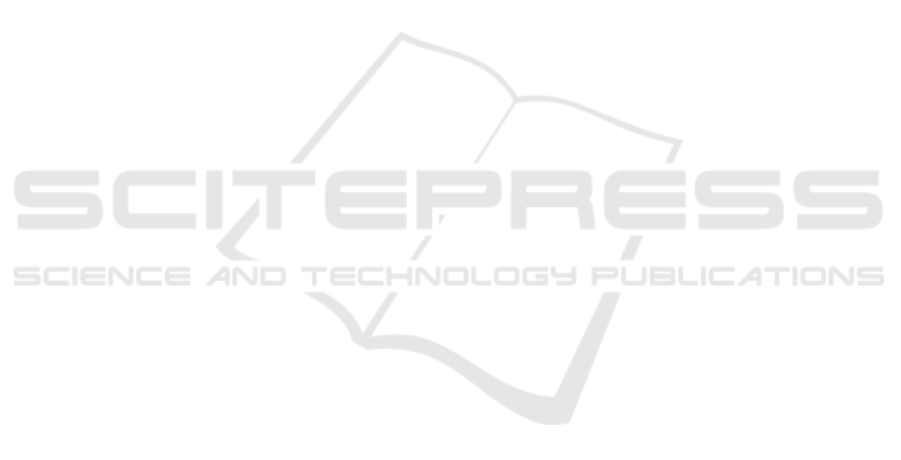
with 4-QAM modulation. The impact is assessed for
different skew between the signals transmitted in the
interfered and interfering cores, different time mis-
alignments and by varying the signals roll-off factors.
To complement our results, the variance of the detec-
ted ICXT is also studied.
Our results show that the roll-off factor of the RC
pulse shape and the skew has a significant influence
on the variance of the detected ICXT. Considering a
null roll-off factor, the variance of the detected ICXT
is constant as a function of the skew. In presence
of skew and with a roll-off factor higher than 0, the
variance of the detected ICXT signal decreases with
the increase of the roll-off factor. For a roll-off factor
higher than 0 and a skew of 25 ps, the variance of the
ICXT reaches its lowest value.
The temporal misalignment has also influence on
the variance of the ICXT signal at the coherent re-
ceiver. Our results reveal that for a given skew, the
variation of the temporal misalignment leads to a ”si-
nusoidal” behavior of the detected ICXT variance.
When the skew is reduced, the difference between
the highest and the lowest variance becomes smal-
ler. Without skew, our results reveal that the OSNR
penalty is independent of the roll-off factor and the 1
dB OSNR penalty is reached with a −16.7 dB ICXT
level. With skew between the cores, the ICXT level
that leads to a 1 dB OSNR penalty is higher for skew
of 25 ps: for β = 0, the ICXT level is −16.7 dB; for
β = 1, the ICXT level for the same OSNR penalty is
−15 dB, due to the reduction of the ICXT variance.
ACKNOWLEDGEMENTS
This work was supported in part by Fundac¸
˜
ao para
a Ci
ˆ
encia e a Tecnologia (FCT) from Portugal un-
der the project of Instituto de Telecomunicac¸
˜
oes
AMEN-UID/EEA/50008/2013 and the ISCTE-IUL
Merit Scholarship BM-ISCTE-2016.
REFERENCES
Agrawal, G. (2010). Fiber-Optic Communication Systems.
John Wiley & Sons, 4 edition.
Carlson, A. and Crilly, P. (2009). Communication Systems.
McGraw-Hill, Boston, 5. ed edition.
Cartaxo, A. and Alves, T. (2017). Discrete Changes Model
of Inter-core Crosstalk of Real Homogeneous Multi-
core Fibers. J. Lightwave Technol., 35(12):2398–
2408.
Cartaxo, A. et al. (2016). Dispersion Impact on
the Crosstalk Amplitude Response of Homogeneous
Multi-Core Fibers. IEEE Photon. Technol. Lett.,
28(17):1858–1861.
Essiambre, R. et al. (2010). Capacity limits of optical fiber
networks. J. Lightwave Technol., 28(4):662–701.
Fini, J. M. et al. (2010). Statistics of Crosstalk in Bent Mul-
ticore Fibers. Opt. Express, 18(14):15122–15129.
Hayashi, T. et al. (2011). Design and Fabrication of Ultra-
Low Crosstalk and Low-Loss Multi-core Fiber. Opt.
Express, 19(17):16576–16592.
Hayashi, T. et al. (2013). Physical Interpretation of Inter-
core Crosstalk in Multicore Fiber: Effects of Mac-
robend, Structure Fluctuation, and Microbend. Opt.
Express, 21(5):5401.
Hayashi, T., Sasaki, T., and Sasaoka, E. (2014). Behavior
of Inter-Core Crosstalk as a Noise and Its Effect on Q
-Factor in Multi-Core Fiber. IEICE Trans. Commun,
(5):936–944.
Hui, R. and O’Sullivan, M. (2008). Fiber Optic Measure-
ment Techniques. Elsevier Academic Press.
Jeruchim, M., Balaban, P., and Shanmugan, K. (2000). Sim-
ulation of communication systems: modeling, method-
ology and techniques. Kluwer Academic Publishers,
Norwell, MA, 2nd edition.
Klaus, W. et al. (2017). Advanced Space Division Multi-
plexing Technologies for Optical Networks. J. Opt.
Commun. Netw., 9(4):C1–C11.
Morioka, T. (2017). High-Capacity Transmission Using
High-Density Multicore Fiber. In Optical Fiber Com-
munication Conference, page Th1C.3.
Puttnam, B. J. et al. (2015). 2.15 Pb/s Transmission Us-
ing a 22 Core Homogeneous Single-Mode Multi-Core
Fiber and Wideband Optical Comb. In European Con-
ference on Optical Communication (ECOC), pages 1–
3.
Puttnam, B. J. et al. (2017). High Capacity Transmission
Systems Using Homogeneous Multi-Core Fibers. J.
Lightwave Technol., 35(6):1157–1167.
Rademacher, G. et al. (2017a). Crosstalk Dynamics in
Multi-Core Fibers. In Opt. Express, pages 12020–
12028.
Rademacher, G. et al. (2017b). Time-Dependent Crosstalk
from Multiple Cores in a Homogeneous Multi-Core
Fiber. In Optical Fiber Communications Conference
and Exhibition (OFC), pages 1–3.
Saitoh, K. and Matsuo, S. (2016). Multicore Fiber Techno-
logy. J. Lightwave Technol., 34(1):55–66.
Takara, H. et al. (2012). 1.01-Pb/s (12 SDM/222 WDM/456
Gb/s) Crosstalk-managed Transmission with 91.4-
b/s/Hz Aggregate Spectral Efficiency. In European
Conference and Exhibition on Optical Communica-
tion (ECOC), page Th.3.C.1.
Xia, T. and Wellbrock, G. (2013). Commercial 100-Gbit/s
Coherent Transmission Systems. In Optical Fiber
Telecommunications, chapter 2, pages 45 – 82. Aca-
demic Press, Boston, 6 edition.
Zhu, B. et al. (2011). 112-Tb/s Space-Division Multiplexed
DWDM transmission with 14-b/s/Hz Aggregate Spec-
tral Efficiency over a 76.8-km Seven-Core Fiber. Opt.
Express, 19(17):16665–16671.
Impact of Inter-core Crosstalk on the Performance of Multi-core Fibers-based SDM Systems with Coherent Detection
81