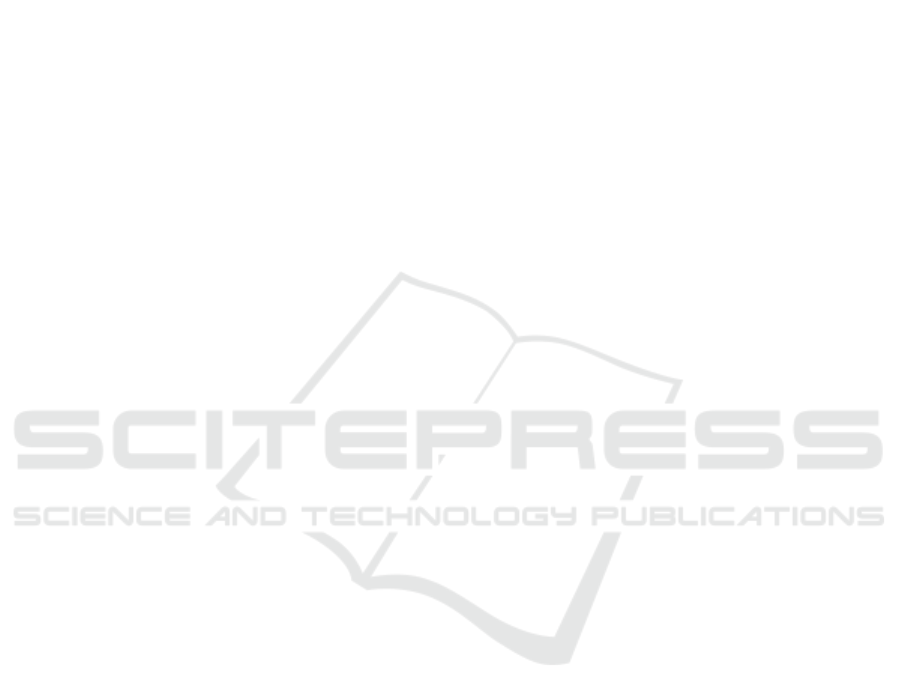
Identifying the Impact of Human Made Transformations of
Historical Watercourses and Flood Risk
Thomas Moran
1
, Sivaraj Valappil
1
and David Harding
2
1
Waste Innovation – Thames Water Utilities Limited, Island Road, Reading, U.K.
2
Waste Planning & Optimisation – Thames Water Utilities Limited, Island Road, Reading, U.K.
Keywords: Geographical Information System, Lidar, Culvert, Watercourse, Digital Terrain Model, Sewer, Flood.
Abstract: In the past, many urban rivers were piped and buried either to simplify development, hide pollution or in an
attempt to reduce flood risk and these factors define a culverted watercourse. A large amount of these
watercourses are not mapped, and if they are, then their original nature is not clearly identifiable due to being
recorded as part of the sewer network. Where these culverted watercourses are not mapped due to being lost
to time and development, we expressed these to be so-called ‘lost rivers’. There is a lack of awareness of the
flood risk in catchments housing these rivers, and because many of them are incorrectly mapped as sewers,
there is often confusion over their legal status and responsibility for their maintenance. To identify the
culverted watercourses many datasets were used including LiDAR data (Ground Elevation Data), historical
maps (earliest 1840's), asset data (Sewer network), and the river network. Automatic and manual identification
of potential culverted watercourses were carried out and then the mapped assets are analysed with flooding
data to understand the impacts. A GIS map has been created showing all potential lost rivers and sites of
culverted watercourses in the North London area.
1 INTRODUCTION
London has a large legacy population of culverted
and concealed watercourses, dating from the 19th and
20th centuries. Since these structures were built,
changes to the governance of drainage have resulted
in many assets being transferred between authorities
and in the process, comprehensive records have not
always survived. There is often uncertainty
surrounding the legal status and responsibility for the
maintenance of culverts. For example, many
culverted watercourses in London were included on
the map of public sewers, where their original status
has become obscured over time. This can be a
significant obstacle to the proper stewardship of the
structures. In addition, the culverting of watercourses
causes problems such as increasing upstream flood
risk due to blockages, reduced ecological value
within concrete channels and with reduced daylight
and adverse effects on environmental features and
wildlife. The issues are summarised as:
Inadequate maintenance and investment – the
responsibility for drainage assets varies according to
their legal status. For example responsibility for a
watercourse normally rests with the owners of land
through which it flows (riparian owners). Where a
watercourse is incorrectly mapped or not mapped at
all, owners may be unaware of their responsibilities.
Different agencies often assume that others are
responsible for such assets, and as a result appropriate
maintenance regimes are not in place. Many of these
assets are critical structures with a high impact of
failure. They should be subject to regular inspection
and have adequate investment plans for their
maintenance and eventual replacement. More
immediately, culverts often have grilles at their inlets
and outlets which can become easily blocked, or
debris causes a blockage within the culvert,
potentially leading to flooding.
Poor understanding of flood risk – culverted
sections of watercourse may drain large, upstream
catchments that extend far beyond the urban area.
Such a situation may not be clear from drainage
records and if it is not appreciated, can lead to
understatement of the flood risk as well as concealing
potential upstream solutions to flooding. A recent
study on drainage capacity relating to the surface
water drainage system around the Mill Hill Circus
junction in London by Transport for London (TfL) is
a good example of this. During periods of medium to
heavy rainfall, the Mill Hill Circus roundabout floods
Moran, T., Valappil, S. and Harding, D.
Identifying the Impact of Human Made Transformations of Historical Watercourses and Flood Risk.
DOI: 10.5220/0006672701730179
In Proceedings of the 4th International Conference on Geographical Information Systems Theory, Applications and Management (GISTAM 2018), pages 173-179
ISBN: 978-989-758-294-3
Copyright
c
2019 by SCITEPRESS – Science and Technology Publications, Lda. All rights reserved
173