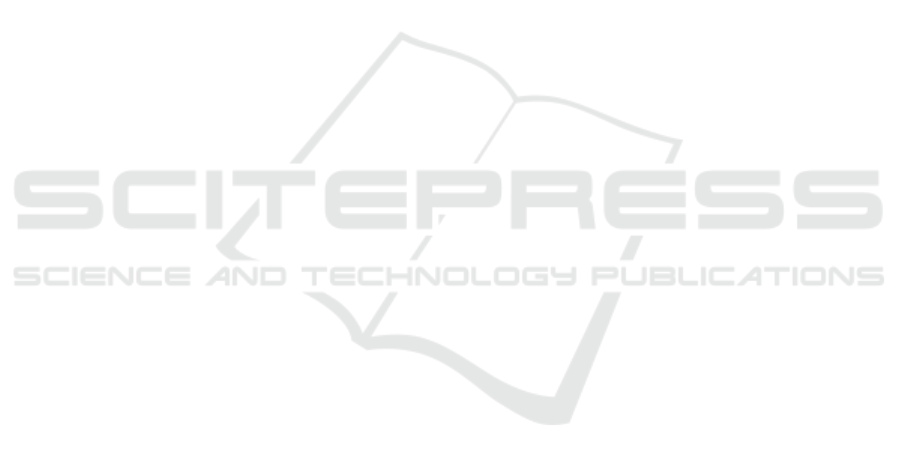
a tunability of 35 nm between 1917-1951 nm, with a
maximum power of 3.84 W at 1934 nm wavelength.
The use of Etalon plates allowed us to reach a
narrow bandwidth of ~0.15 nm.
Comparing to previous work done with Tm:YAP
lasers, we report a first Watt level tunable narrow
bandwidth laser. Tm:YAP with the b-cut orientation,
which emits around the water absorption peak at
1940 nm, could be applicable for tissues ablation
with a controlled penetration depth, besides the other
applications of tunable source in the 2 μm region.
ACKNOWLEDGEMENTS
The authors would like to thank Y. Sebbag and
Prof. U. Levy from the Hebrew University of
Jerusalem, for there willingness and support, and to
D. Sebbag and R. Nahir for his fruitful discussion
and collaboration.
REFERENCES
Coluccelli, N., Galzerano, G., Laporta, P., Cornacchia, F.,
Parisi, D. and Tonelli, M. (2007). Tm-doped LiLuF_4
crystal for efficient laser action in the wavelength
range from 182 to 206 μm. Optics Letters, 32(14),
p.2040.
Eremeikin, O., Savikin, A., Pavlenko, K. and Sharkov, V.
(2010). Diode-pumped tunable Tm:YLF laser for mid-
infrared gas spectroscopy. Quantum Electronics,
40(6), pp.471-474.
Feng, T., Yang, K., Zhao, S., Zhao, J., Qiao, W., Li, T.,
Zheng, L. and Xu, J. (2014). Broadly wavelength
tunable acousto-optically Q-switched Tm:Lu2SiO5
laser. Applied Optics, 53(27), pp.6119-6122.
Feng, T., Zhao, S., Yang, K., Li, G., Li, D., Zhao, J., Qiao,
W., Hou, J., Yang, Y., He, J., Zheng, L., Wang, Q.,
Xu, X., Su, L. and Xu, J. (2013). Diode-pumped
continuous wave tunable and graphene Q-switched
Tm:LSO lasers. Optics Express, 21(21), pp.24665-
24673.
Godard, A. (2007). Infrared (2–12 μm) solid-state laser
sources: a review. Comptes Rendus Physique, 8(10),
pp.1100-1128.
Koechner, W. (2006). Solid-State Laser Engineering. New
York (NY): Springer.
Li, J., Yang, S., Zhang, H., Hu, D. and Zhao, C. (2010).
Diode-pumped room temperature single frequency
Tm:YAP laser. Laser Physics Letters, 7(3), pp.203-
205.
Li, J., Yang, S., Zhao, C., Zhang, H. and Xie, W. (2010).
High efficient single-frequency output at 1991 nm
from a diode-pumped Tm:YAP coupled cavity. Optics
Express, 18(12), pp.12161-12167.
Li, G., Liu, H., Lu, F., Wen, X., Gu, Y. and Wang, Y.
(2014). Analysis on preferential free running laser
wavelength and performance modeling of Tm3+-
doped YAP and YLF. Applied Optics, 53(22), p.4987.
Scholle, K., Fuhrberg, P., Koopmann, P. and Lamrini, S.
(2010). 2 µm Laser Sources and Their Possible
Applications. INTECH Open Access Publisher.
Sorokina, I. (2003). Crystalline Mid-Infrared Lasers.
Topics in Applied Physics, 89, pp.262-358.
Sun, M., Long, J., Li, X., Liu, Y., Ma, H., An, Y., Hu, X.,
Wang, Y., Li, C. and Shen, D. (2012). Widely tunable
Tm:LuYAG laser with a volume Bragg grating. Laser
Physics Letters, 9(8), pp.553-556.
Wang, L., Gao, C., Gao, M., Liu, L. and Yue, F. (2013).
Diode-pumped 2 μm tunable single-frequency
Tm:LuAG laser with intracavity Etalons. Applied
Optics, 52(6), pp.1272-1275.
Yu-Feng, L., You-Lun, J., Bao-Quan, Y., Yue-Zhu, W.
and Ubizskii, S. (2007). A Laser-Diode-Pumped
Widely Tunable Single-Longitude-Mode Tm:YAP
Laser at Room Temperature. Chinese Physics Letters,
24(9), pp.2594-2596.
Narrow Bandwidth Tunable Watt Level Tm:YAP Laser using Two Etalons
307