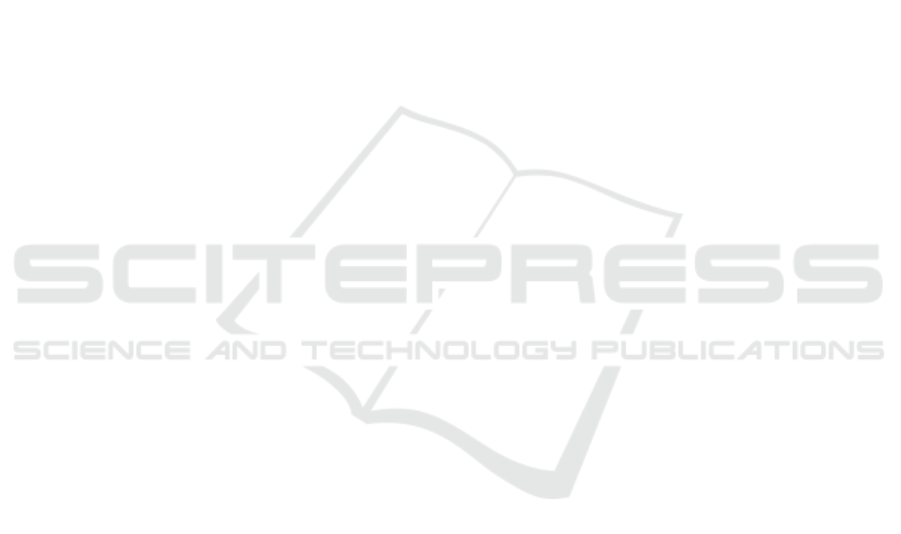
Competitividade—COMPETE and Fundação para a
Ciência e a Tecnologia—FCT, by the project
PTDC/FIS-NAN/0909/2014 and for the Portuguese
research Grants No. PEst-OE/FIS/UI0068/2011 and
UID/FIS/00068/2013. Telma Marques and Filipa
Pires acknowledge the fellowships
SFRH/BD/106032/2015 and PD/BD/106036/2015,
respectively from RABBIT Doctoral Programme
(RaBBiT, PD/00193/2012), Portugal.
REFERENCES
Bernhardt, J. H., 1992. Non-ionizing radiation safety:
Radiofrequency radiation, electric and magnetic fields.
Phys. Med. Biol. 37,807-844.
Bronzino, J.D., 1995. The biomedical engineering
handbook. CRC Press & IEEE Press. 2nd edition.
Caruso, F., Möhwald, H., 1999. Protein Multilayer
Formation on Colloids through a Stepwise Self-
Assembly Technique. Contribution from the Max
Planck Institute of Colloids and Interfaces, D-14424
Potsdam, Germany. J. Am. Chem. Soc., 1999, 121 (25),
6039–6046.
Chen, R.Z., Craik, S.A., Bolton, J.R. 2009. Comparison of
the action spectra and relative DNA absorbance spectra
of microorganisms: Information important for the
determination of germicidal fluence (UV dose) in an
ultraviolet disinfection of water. Water Research.
43(20):5087-96.
Franklin, R.E., Gosling, R.G., 1953. Evidence for 2-Chain
Helix in Crystalline Structure of Sodium
Deoxyribonucleate. Nature, 172, 156.
Giovanetti, A., Sgura, A., Aversa, G., 2012. Biological
Dosimetry - How to Measure the Absorbed Dose in
Different Scenarios. ENEA - Italian national agency for
new technologies, energy and sustainable economic
development. Rome. ISBN: 978-88-8286-264-0.
Gomes, P.J., 2014. Characterization of molecular damage
induced by UV photons and carbon ions on biomimetic
heterostructures. PhD Dissertation, FCT/UNL,
Portugal.
Gomes, P. J., Coelho, M., Dionísio, M., Ribeiro, P.A.,
Raposo, M., 2012. Probing radiation damage by
alternated current conductivity as a method to
characterize electron hopping conduction in DNA
molecules. Applied Physics Letters, 101, 123702.
Gomes, P.J., Ferraria, A. M., Botelho do Rego, A. M.,
Hoffmann, S.V., Ribeiro, P.A., Raposo, M., 2015.
Energy Thresholds of DNA Damage Induced by UV
Radiation: An XPS Study. The journal of Physical
Chemistry B, 119 (17) 5404- 5411.
Gomes, P.J., Ribeiro, P.A., Shaw, D., Mason, N.J. and
Raposo, M., 2009. UV degradation of deoxyribonucleic
acid. Polymer Degradation and Stability, 94(12) pp.
2134–2141.
Kielbassa, C., Roza, L., Epe, B., 1997. Wavelength
dependence of oxidative DNA damage induced by UV
and visible light. Carcinogenesis, 18, 811.
Lvov, Y., Decher, G., Sukhorukov, G., 1993. Assembly of
thin films by means of successive deposition of
alternate layers of DNA and poly(allylamine).
Macromolecules. 26 (20), pp 5396–5399.
Moulder, J., 2007. Power Lines, Cancer FAQ’s.
Electromagnetic Fields and Human Health. J.E. USA.
National Research Council, 1995. Chapter 6 - Biologic
Dosimetry and Biologic Markers: Growing public
concern about. Radiation Dose Reconstruction for
Epidemiologic Uses. Washington, DC: The National
Academies Press. ISBN: 978-0-309-05099-9.
Podgorsak, E.B., 2006. Radiation Physics for Medical
Physicist. Springer, Heidelberg. ISBN 978-3-642-
00875-7.
Raposo, M., Pontes, R.S., Mattoso, L.H.C., Oliveira
O.N.Jr., 1997. Kinetics of Adsorption of Poly (o-
methoxyaniline) Self-assembled films.
Macromolecules, 30, 6095-6101.
Ravanat, J.L., Douki, T., 2016., UV and ionizing radiations
induced DNA damage, differences and similarities.
Radiat. Phys. Chem.
https://doi.org/10.1016/j.radphyschem.2016.07.000.
Ravanat, J.L., Douki, T. and Cadet, J., 2001. Direct and
Indirect Effects of UV Radiation on DNA and Its
Components. Journal of Photochemistry and
Photobiology B: Biology, 63, 1-3, 88-102.
Schuch A.P., Garcia C.C., Makita K., Menck C.F., 2013.
DNA damage as a biological sensor for environmental
sunlight. Photochem. Photobiol. Sci., 2013, 12, 1259-
1272.
Su, H., Kallury, K.M.R., Thompson, M., Roach, A., 1994.
Interfacial Nucleic Acid Hybridization Studied by
Random Primer 32P Labeling and Liquid-Phase
Acoustic Network Analysis. Anal.Chem. 66, 769.
Vincent, W.F., Neale, P.J., 2000. 6 - Mechanisms of UV
damage to aquatic organisms. The Effects of UV
Radiation in the Marine Environment. Cambridge
University Press.
Wilkins, M.H., Stokes, A.R., Wilson, H.R., 1953.
Molecular Structure of Nucleic Acids: Molecular
Structure of Deoxypentose Nucleic Acids. Nature, 171,
738.
Xu, X.H., Yang, H.C., Mallouk, T.E., Bard, A.J., 1994.
Immobilization of DNA on an Aluminum (III)
Alkanebisphosphonate Thin Film with
Electrogenerated Chemiluminescent Detection.
J.Am.Chem.Soc. 116, 8386.
Yu, S.L., Lee, S.K., 2017. Ultraviolet radiation: DNA
damage, repair, and human disorders. Mol Cell Toxicol,
13:21-28.
Development of a DNA Biodosimeter for UV Radiation
333