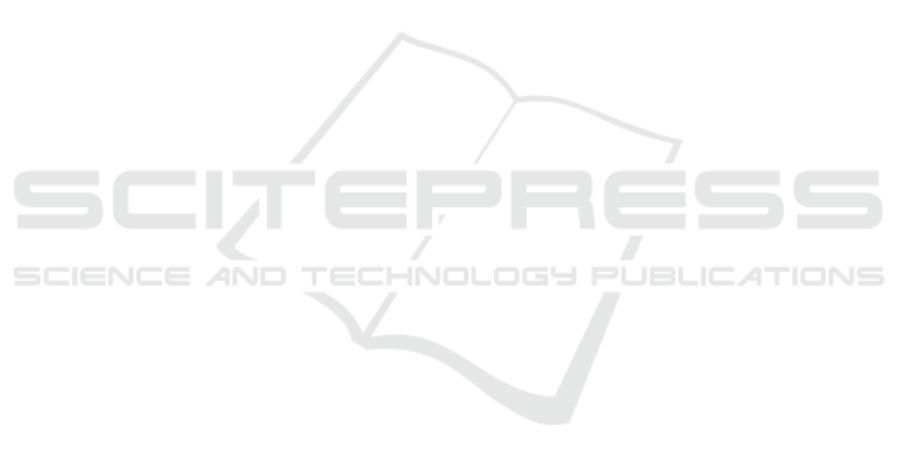
As can be seen by Figure 6 analysis all the
materials tested demonstrated to be catalytic in this
photodegradation process. Without any catalyst,
after 60 min of irradiation, less than 10% of Rh6G,
were degraded. On the other hand, in the presence of
WO
3
, TiO
2
, TiO
2
/WO
3
and WO
3
/TiO
2
after 60 min
of irradiation approximately 80, 20, 65 and 45% of
Rh6G, were degraded respectively. These results
reveal that the WO
3
film exhibit the best
photocatalytic performance, followed by the
TiO
2
/WO
3
bilayered film. However, the WO
3
film
suffers of low mechanical stability, which isn’t
observed for the bilayered films independently of the
upper layer. It is well known that the materials
catalytic performance results from the balance of
several factors such as the surface area, crystal
structure, adsorption activity, band gap and so on. In
this work and analyzing the band gap values
obtained for these oxides the catalytic results point
out that the lower band gap obtained for the WO
3
films and also for the bilayered films seems to be a
preponderant factor on the films photocatalytic
activity. It is further observed that in the case of the
bilayered films, when the upper layer is WO
3
the
photocatalytic activity is higher than the one
observed for the other bilayered film when the upper
layer is TiO
2
. This difference in the catalytic activity
may be attributed to the different surface
morphology observed for these two types of
bilayered films.
4 CONCLUSIONS
TiO
2
/WO
3
and WO
3
/TiO
2
bilayered films were
deposited on glass substrates by DC-reactive
magnetron sputtering. The bilayered films exhibit
good adherence to the substrates and high mechanic
stability. In both composites independently of the
above layer, the main phase observed in the X-ray
patterns corresponds to the WO
3
. The bilayered
films and in particular with WO
3
as the upper layer
exhibit high catalytic performance for Rh6G
degradation under visible irradiation which can be
mainly attributed to the lower band gap in
comparison with TiO
2
and also to the surface
morphology. These findings are encouraging for
future studies on the improvement of bilayered films
photocatalytic efficiency and promote its practical
application in environmental remediation using solar
irradiation.
ACKNOWLEDGEMENTS
The authors acknowledge the financial support from
FEDER, through Programa Operacional Factores de
Competitividade − COMPETE and Fundação para a
Ciência e a Tecnologia − FCT, for the projects
UID/MULTI/00612/2013 and UID/FIS/00068/2013.
REFERENCES
Akhavan, O., Azimirad, R., Appl. Catal., A, 369,77, 2009.
Akhavan, O., Appl. Surf. Sci., 257, 1724, 2010.
Barrocas, B., Monteiro, O. C., Melo Jorge, M. E., Sério,
S., Appl. Surf. Sci., 264, 111−116, 2013.
Barrocas, B., Sério,S., Rovisco, A., Melo Jorge, M.E., J.
Phys. Chem. C, 118, 590−597, 2014.
Celik, E., Yildiz, A. Y., Ak Azem, N. F., Tanoglu, M.,
Toparli, M., Emrullahoglu, O.F., Ozdemir, I., Mater.
Sci. Eng., B, 129, 193, 2006.
Crini, G., Badot, P. M. Progress in polymer science, 33(4),
399-447, 2008.
Das, S., Kamat, P.V., Padmaja, S., Au, V., Madison, S. A.,
J. Chem. Soc., Perkins Trans., 2, 1219-1224, 1999.
Diebold, U., Surf. Sci. Rep., 578, 48, 53−229, 2003.
Fernandez-Ibanez, P., Blanco, J., Malato, S., de las
Nieves, F.J., Water Res., 37, 3180–3188, 2003.
González-Borrero, P. P., Sato, F., Medina, A. N., Baesso,
M. L., Bento, A. C., Baldissera, G., Persson, C.,
Niklasson, G. A., Granqvist, C. G., Ferreira da Silva,
A., Appl. Phys. Lett., 96, 061909, 2010.
Grätzel, M., Nature, 414, 338, 2001.
Jimenez, I., Arbiol, J., Dezanneau, G., Cornet, A.,
Morante, J. R., Sens. Actuators, B, 93, 475, 2003.
Kavan, L., Grätzel, M., Electrochim. Acta, 40, 643, 1995.
Keller, V., Bernhardt, P., Garin, F., J. Catal., 215, 129,
2003.
Krishnamoorthy, S., Baker, J. P., Amiridis, M. D., Catal.
Today, 40, 39, 1998.
Lin, C. F., Wu, C. H.,. Onna, Z. N, J. Hazard. Mater, 154,
1033, 2008.
Marci, G., Augugliaro, V., López-Munoz, M. J., Martín,
C., Palmisano, L., Rives, V., Schiavello, M.,
Tilleyand, R. J., Venezia, A. M., J. Phys. Chem. B,
105, 1033, 2001.
Marsen, B., Cole, B., Miller, E. L., Sol. Energy Mater.
Sol. Cells, 91, 1954, 2007.
Mills, A., Hunte S. Le, J. Photochem. Photobiol., A, 108,
1, 1997.
Naidu, S. V. N., Rao, P. R., Phase Diagrams of Binary
Tungsten Alloys, Indian Institute of Metals, Calcutta,
1991.
Nova, I., Lietti, L., Tronconi, E., Forzatti, P.,Catal. Today,
60, 73, 2000.
Ozmen, E. Y., Erdemir, S., Yilmaz, M., Bahadir, M.,
Clean: Soil, Air, Water 35, 612−616, 2007.
Roy Choudhury, A.K., Environmental Impacts of the
Textile industry and Its Assessment Through Life
Photocatalytic Degradation of Rhodamine 6G using TiO
2
/WO
3
Bilayered Films Produced by Reactive Sputtering
339