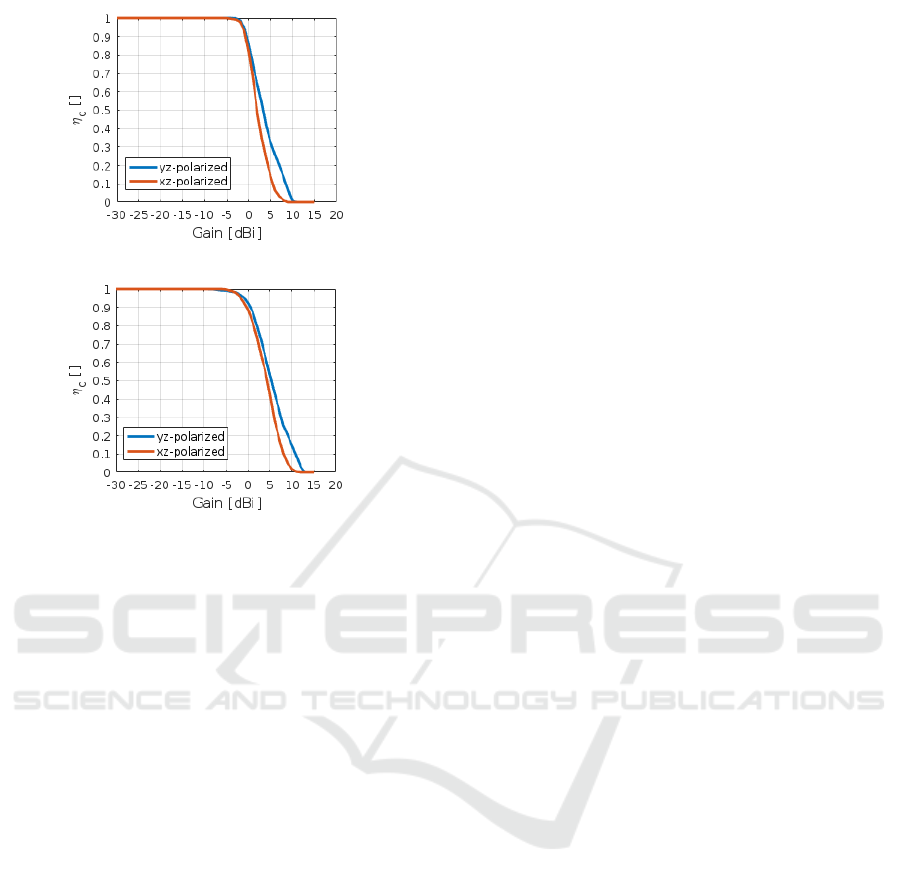
(a)
(b)
Figure 10: Coverage efficiency of phased antenna array in
(a) configuration 1 and (b) configuration 2.
Finally, the coverage efficiency of the array in two
configurations is calculated and shown in Figure 10.
First, it can be noticed that difference in the coverage
between two polarization is very small for the gains
lower than 0 dBi. However, a bigger difference can
be seen as the gain increases. Finally, the curves for
the array configuration 1 and configuration 2 have a
very similar slope, but the absolute level of the cov-
erage is different. It can be seen that the array in the
configuration 1 have 20 % less coverage for the gain
of 5 dBi.
4 CONCLUSION
In this paper, a dual-polarized dual-band phased an-
tenna array for 5G mobile devices has been presented.
The proposed array have the bandwidth of 3.6 GHz
and covers the band of 30.8 to 33.4 GHz. Further-
more, it has been shown how to tune each mode of the
antenna in order to achieve the desired bandwidth and
resonance frequency of each mode. Next, two phased
array configurations have been investigated. One con-
figuration has 8 array elements distributed into two
4-element sub-arrays oriented orthogonally with re-
spect to each other. It has been found that array of
8 elements give better spatial coverage performance.
However, this investigation has only been done for the
broadside antenna element. A further investigation of
endfire antenna element should also be conducted in
the future work.
REFERENCES
Helander, J., Zhao, K., Ying, Z. & Sjöberg, D. (2016), ‘Per-
formance analysis of millimeter-wave phased array
antennas in cellular handsets’, IEEE Antenna Wireless
Propagation Letters 15, 504–507.
Hong, W., Baek, K., Lee, Y. & Kim, Y. G. (2014), ‘Design
and analysis of a low-profile 28 GHz beam steering
antenna solution for Future 5G cellular applications’,
Microwave Symposium (IMS), 2014 IEEE MTT-S In-
ternational pp. 1–4.
Hong, W., Ko, S. T., Lee, Y. & Baek, K. H. (2015), ‘Multi-
polarized antenna array configuration for mmWave
5G mobile terminals’, 2015 International Workshop
on Antenna Technology (iWAT) pp. 60–61.
Hussain, R., Alreshaid, A. T., Podilchak, S. K. & Sharawi,
M. S. (2017), ‘Compact 4G MIMO antenna inte-
grated with a 5G array for current and future mobile
handsets’, IET Microwaves, Antennas & Propagation
11(2), 271–279.
Lee, J., Tejedor, E., Ranta-aho, K., Wang, H., Lee, K. T.,
Semaan, E., Mohyeldin, E., Song, J., Bergljung, C. &
Jung, S. (2018), ‘Spectrum for 5g: Global status, chal-
lenges, and enabling technologies’, IEEE Communi-
cation Magazine 56(3), 12–18.
Mahmoud, K. R. & Montaser, A. M. (2018), ‘Design of
dual-band circularly polarised array antenna pack-
age for 5G mobile terminals with beam-steering ca-
pabilities’, IET Microwaves, Antennas Propagation
12(1), 29–39.
Nielsen, J. O. & Pedersen, G. F. (2016), Dual-polarized in-
door propagation at 26 ghz, in ‘2016 IEEE 27th An-
nual International Symposium on Personal, Indoor,
and Mobile Radio Communications (PIMRC)’, pp. 1–
6.
Ojaroudiparchin, N., Shen, M. & Pedersen, G. F. (2015),
‘Design of Vivaldi antenna array with end-fire beam
steering function for 5G mobile terminals’, 2015
23rd Telecommunications Forum Telfor (TELFOR)
pp. 587–590.
Ojaroudiparchin, N., Shen, M., Zhang, S. & Pedersen, G. F.
(2016), ‘A Switchable 3-D-Coverage-Phased Array
Antenna Package for 5G Mobile Terminals’, IEEE
Antennas Wireless Propagation Letters 15, 1747–
1750.
Rappaport, T. S., Sun, S., Mayzus, R., Zhao, H., Azar, Y.,
Wang, K., Wong, G. N., Schulz, J. K., Samimi, M. &
Gutierrez, F. (2013), ‘Millimeter wave mobile com-
munications for 5G cellular: It will work!’, IEEE Ac-
cess 1, 335–349.
Rehman, M. U., Chen, X., Parini, C. G. & Ying, Z. (2012),
‘Evaluation of a statistical model for the characteriza-
tion of multipath affecting mobile terminal GPS an-
tennas in sub-urban areas’, IEEE Transactions on An-
tennas and Propagation 60(2), 1084–1094.
WINSYS 2018 - International Conference on Wireless Networks and Mobile Systems
314