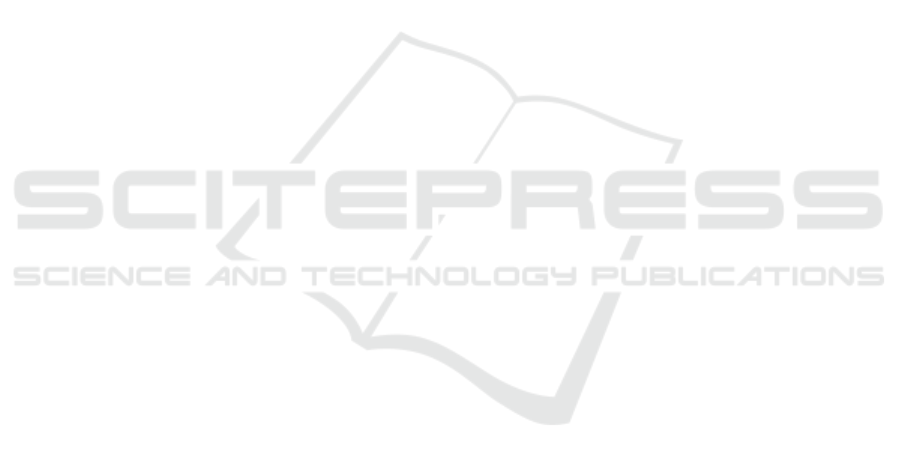
REFERENCES
Alkozei A., Smith R., Pisner D.A, Vanuk J.R, Berryhill
S.M, Fridman A., Shane B.R., Knight S.A., Killgore
W.D.S., 2016. Exposure to blue light increases
subsequent functional activation of the prefrontal
cortex during performance of a working memory task.
Sleep, 39 (9), 1671-1680. https://doi.org/10.5665/
sleep.6090
Baek, H. Min, B.K., 2015. Blue light aids in coping with
the post-lunch dip: an EEG study. Ergonomics, 58 (5),
803-810.
Bellia L., Bisegna F., Spada G., 2011. Lighting in indoor
environments: Visual and non-visual effects of light
sources with different spectral power distributions.
Build Environ 46, 1984-1992. https://doi.org/10.1016/
j.buildenv.2011.04.007
Cajochen C., 2007. Alerting effects of light. Sleep Med
Rev., 11, 453-464.
Cajochen C., Chellappa S., Schmidt C., 2010. What keeps
us awake? The role of clocks and hourglasses, light,
and melatonin. Int Rev Neurobiol, 93, 57-90.
Chang A.M., Scheer F.A.J, Czeisler C.A., Aeschbach D.,
2013. Direct effects of light o alertness, vigilance, and
the waking electroencephalogram in humans depend
on prior light history. Sleep, 36 (8), 1239-1246.
Chellappa S.L., Steiner R., Blattner P., Oelhafen P., Gotz
T., Cajochen C., 2011. Non-visual effects of light on
melatonin, alertness and cognitive performance: can
blue enriched light keep us alert? PLoS One, 6,
e16429.
Figueiro M.G., 2013. Non-Visual Lighting Effects and
Their Impact on Health and Well-Being. In
Encyclopedia of Color Science and Technology.
Springer Science+Business Media New York doi:
10.1007/978-3-642-27851-8_118-4, 1-11.
Figueiro M.G., Bierman A., Plitnick B., Rea M.S., 2009.
Preliminary evidence that both blue and red light can
induce alertness at night. BMC Neurosci, 10. 10:105.
https://doi.org/10.1186/1471-2202-10-105
Figueiro M.G., Rea M.S., 2010. The effects of red and
blue light on circadian variations in cortisol, alpha
amylase and melatonin. Int J Endocrinol., Volume
2010, Article ID 829351, 9 pages, https://doi.org/
10.1155/2010/829351
Figueiro M.G., Rea M.S., 2017. Quantifying circadian
light and its impact. Architectural lighting
http://www.archlighting.com/technology/quantifying-
circadian-light-and-its-impact_o (retrieved 018/04/02).
Figueiro M.G., Sahin, L., Wood, B., Plitnick, B., 2016.
Light at night and measures of alertness and
performance: implications for shift workers. Biol Res
Nurs.. 18 (1), 90-100. https://doi.org/10.1177/
1099800415572873
Jankowski K.S., 2015. Composite Scale of Morningness:
psychometric properties, validity with Munich
ChronoType Questionnare and age/sex differences in
Poland. Eur Psychiatry, 30, 166-171.
Klimesch W., 2012. Alpha-band oscillations, attention,
and controlled access to stored information. Trends
Cogn Sci. 16 (12), 606-617.
Łaszewska K., Goroncy A., Weber P., Pracki T., Tafil-
Klawe M., Pracka D., Złomańczuk P., 2017. Daytime
acute non-visual alerting response in brain activity
occurs as a result of short- and long wavelengths of
light. Journal of psychophysiology. https://doi.org/
10.1027/0269-8803/a000199
Maierova L., Borisuit A., Scartezzini J.L., Jaeggi S.M.,
Schmidt C., Münch M., 2016. Diurnal variations of
hormonal secretion, alertness and cognition in extreme
chronotypes under different lighting conditions.
Nature. Scientific Reports, 1-10. https://doi.org/
10.1038/srep33591
Okamoto Y., Rea M.S., Figueiro M.G., 2014. Temporal
dynamics of EEG activity during short and long
wavelength light exposures in the early morning. BMC
Res Notes, 7: 113, 1-6.
Phipps-Nelson J., Redman J.R., Schlangen L.J.,
Rajaratnam S.M., 2009. Blue light exposure reduces
objective measures of sleepiness during prolonged
night time performance. Chronobiol Int. 26 (5), 891-
912. doi: 10.1080/07420520903044364.
Plitnick B., Figueiro M.G., Wood B., Rea M.S., 2010. The
effects of red and blue light on alertness and mood at
night. Lighting Res. Technol, 42 (4), 449-458.
https://doi.org/10.1177/1477153509360887
Sahin L. and Figueiro M.G., 2013. Alerting effects of
short-wavelength (blue) and long – wavelengths (red)
lights in the afternoon. Physiol Behav. 116-117 (5), 1-
7. https://doi.org/10.1016/j.physbeh.2013.03.014
Sahin L., Wood B.M., Plitick B., Figueiro M.G., 2014.
Daytime light exposure: effects on biomarkers,
measure of alertness and performance. Behav Brain
Res. 274, 176-185. https://doi.org/10.1016/j.bbr. 2014.
08.017
Sawicki, D., Wolska, A., Rosłon, P., Ordysiński, S. 2016.
New EEG Measure of the Alertness Analyzed by
Emotiv EPOC in a Real Working Environment. In
Proc. of the 4th International Congress on
Neurotechnology, Electronics and Informatics,
NEUROTECHNIX 2016, Porto, Portugal, 7-8 Nov.
2016, 35-42. doi: 10.5220/0006041200350042
Smith C.S., Reilly C., Midkiff K., 1989. Evaluation of
three circadian rhythm questionnaires with suggestion
for an improved measure of morningness. J Appl
Psychol., 75, 728-738.
NEUROTECHNIX 2018 - 6th International Congress on Neurotechnology, Electronics and Informatics
60