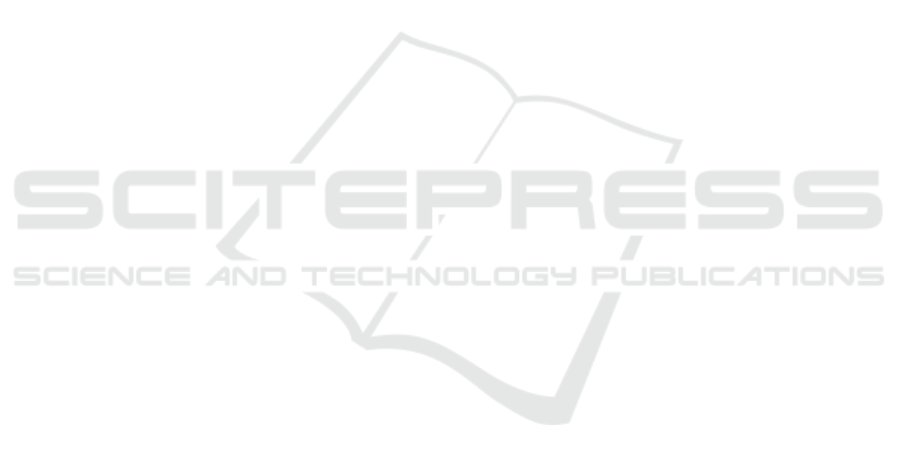
maintenance, such as selecting maintenance strategies and allocating maintenance funds, is
imperative for Australian road authorities.
Road maintenance has been a well-studied research area, especially in using deterioration
modelling techniques to predict the pavement performance [5]. Traditional maintenance management
decisions are based on the deterioration models, as well as the life cycle cost of various maintenance
strategies. For example, four major maintenance strategies, including rehabilitation, overlay,
Microsurfacing and slurry seal are investigated in [5]. A network-level budget allocation program
was also developed to optimize two indicators, including pavement performance improvement and
budget utilization [6]. It should be noted that as sustainability becomes a rising concern, it is
important to understand other aspects of road maintenance, such as the environmental impact of road
maintenance activities and effectively integrate these aspects into the maintenance management
framework.
It is widely acknowledged that maintenance activities should be conducted before road assets
deteriorate to achieve long-term performance. Many studies have therefore been conducted on
evaluating the deterioration of road pavement. Generally, the deterioration models have been well
developed. For example, Markov model has been widely used for deterioration forecasting and
performance under the curve can be used as an indicator of the improvement potential of pavement
strategies [7].
Over the past few years, a few other important indicators to evaluate the effectiveness of
maintenance strategies have also been developed. The environmental impacts of various maintenance
activities can be quite different because the inputs of these activities, such as resources and the use of
equipment, vary significantly. Life cycle assessment, which is a method to evaluate the
environmental outputs of products or processes based on the inputs, can be adopted [8]. A few
commonly adopted system boundaries, i.e. the life cycle stages that are included in the assessment,
are cradle-to-grave, cradle-to-gate and gate-to-gate. Cradle-to-grave refers to the full life cycle stages,
starting from raw materials extraction to final disposal. Cradle-to-gate refers to the life cycle stages,
starting from raw materials extraction to the point when the product leaves the production factory. In
addition, gate-to-gate refers to the life cycle stages within an organization, such as manufacturing
company or supplier. The assessment procedure has four stages, including scope definition, life cycle
inventory analysis to list all inputs (i.e. resources) and outputs (i.e. environmental impacts), impact
assessment to convert inputs to outputs, and interpretation [9].
Eight pavement maintenance strategies are investigated in this paper, including:
ASDG - Dense graded asphalt overlay/replacement. This strategy is related to the use of
asphalt replacement. The activities include asphalt mixing, paving and compacting. The
equipment used in this strategy include asphalt mixing plant, asphalt paver and asphalt
compactor. The depth of the asphalt replacement in this strategy is 30mm.
ASIM – Intersection mix asphalt overlay/replacement. Similar with ASDG, this strategy
is related to the use of asphalt replacement and the depth of the replacement is 40mm.
ASOG – Open graded asphalt replacement. Similar with ASDG, this strategy is related to
the use of asphalt replacement and the depth of the replacement is 30mm.
ASRS – Structural asphalt work. A full depth asphalt (150mm) will be replaced every 50
years and 5% of road placed will need patching and repair every 50 years.
GrOL – Granular overlay. This strategy refers to granular overlay with spray and seal.
RipSeal – RipSeal includes a 150mm cement stabilization, 50mm gravel placement and a
seal of 10mm.
Slurry – Slurry/micro surfacing. This strategy is cold mix surface treatment, including a
3-20mm layer of in-situ mixture of aggregate, bitumen, adhesion agents, water and
cement.
Evaluating the Environmental Impacts of Pavement Maintenance Strategies Based on Life Cycle Assessment
39