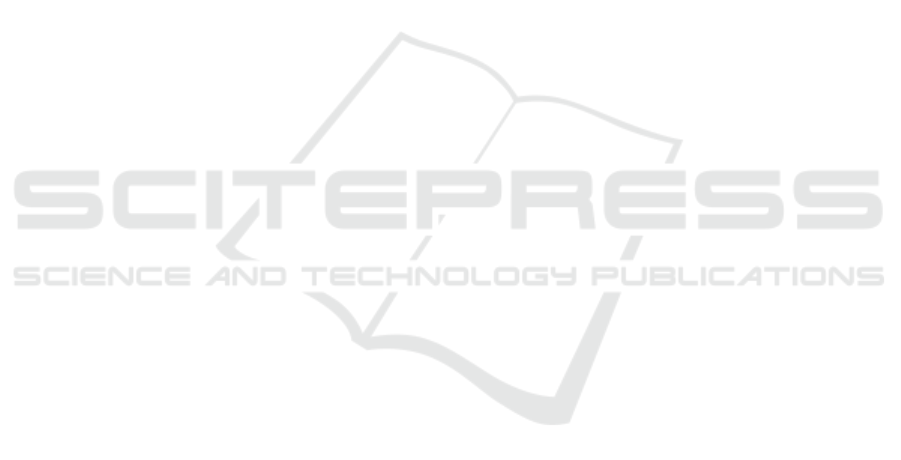
Schiff-base Complexes Immobilized on the Fe
3
O
4
@SiO
2
for
Selective Oxidation of Benzyl Alcohol
F H Li
1
, S S Jia
1
, Y W Fang
1
and Y B Song
1, *
1
College of Science,Shantou University, Shantou, Guangdong, 515063, China
Corresponding author and e-mail: Y B Song, ybsong@stu.edu.cn
Abstract. Efficient and highly selective heterogeneous catalysts were prepared through
grafting the metal-salen on the modified magnetic nanoparticles. Fe
3
O
4
nanoparticles were
prepared was hydrothermal synthesis, after the Fe
3
O
4
@ SiO
2
was amino-functionalized using
3-aminopropyltriethoxysilane and then Fe
3
O
4
@ SiO
2
-NH
2
was obtained by the reaction.
Finally, Fe
3
O
4
@ SiO
2
[(EtO)
3
Si-L
2
]/Mn were successfully synthesized. These
surface-modified nanoparticles were using various characterize techniques such as XRD,
FT-IR and SEM. The heterogeneous catalyst showed high conversion and selectivity in the
reaction of oxidation of benzyl alcohol. Furthermore, the catalysts were easily separated by
the external magnetic field after the reaction, reused at least 8 consecutive cycles without
significant loss catalysis activity.
1. Introduction
Metal Schiff-base complexes have a wide range of applications in catalysis. Schiff-base complexes
coordinate with transition metal easily, and form steadily metal Schiff-base complexes with different
valence state. The metal Schiff-base complexes could catalysis a lot kinds of chemical reactions, and
prepare essential chemical intermediates, such as polymerization reaction [1], oxidation reaction
[2-3], epoxidation reaction [4], reduction reaction [5], Michael addition reaction [6], cyclopropane
reaction [7-8], ring opening reaction [9]. Homogeneous metal Schiff-base complexes have high
catalytic activity. However it is difficult to separate them from the products, they are not conducive to
reuse, which greatly limits the catalyst of reuse. In addition, in the process of the catalysis reaction,
polymerization phenomenon can also lead to deactivation of catalyst. So the heterogenization of the
homogeneous catalyst research increasingly draws people attention. Heterogenization not only can
keep the structure of the homogeneous catalyst and the excellent properties, but also can avoid the
disadvantage of recycling hard. At the same time, Heterogenization could show some synergistic
effect, which can improve the catalyst of performance.
At present, the supporting according to their material can be divided into inorganic supporting,
organic synthetic polymer supporting, natural high polymer supporting. Inorganic supports mainly
including carbon nanotubes, carbon nanofibers, SiO
2
particles, zeolites. These supports with high
specific surface area to disperse the metal Schiff-base complex greatly improve the catalytic
performance. Guangxing Li encapsulated Co(salen) in NaY type molecular sieve to catalyze the
oxidative carbonylation reaction of benzene ammonia under the condition of 170°C , and high
conversion and selectivity were obtained [10]. Priti Sharma grafted Mn Schiff-base complexes on the
modified SBA-15 to catalyze the oxidation of sulfide [11]. Organic synthetic polymer carrier contains
Li, F., Jia, S., Fang, Y. and Song, Y.
Schiff-Base Complexes Immobilized on the Fe3O4@SiO2 for Selective Oxidation of Benzyl Alcohol.
In Proceedings of the International Workshop on Materials, Chemistry and Engineering (IWMCE 2018), pages 409-415
ISBN: 978-989-758-346-9
Copyright © 2018 by SCITEPRESS – Science and Technology Publications, Lda. All rights reserved
409