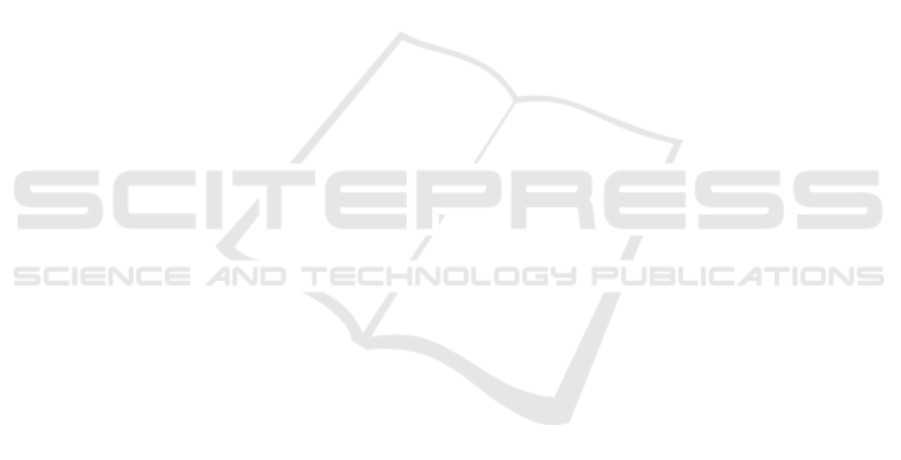
inorganic materials in wood to form wood-inorganic composites to improve the strength and thermal
stability of wood[9]; 3) compression treatment to increase the strength of wood[10]. However, all
these methods have certain fatal defects, and are difficult to make the modified wood being met the
practical requirements. For example, 1) although the strength of the wood-polymer composite is
significantly increased, the impact toughness is far lower than that of unmodified wood, suggesting
brittleness; 2) the strength of wood-inorganic composites are normally less improved and brittle; 3)
the strength of compressed wood are traditionally improved, but the thickness are easy spring-back in
wet condition. Although the dimensional stability could be improved by impregnating formaldehyde-
based resins, such treatment adversely results in significant reduction in the impact toughness of the
modified wood. In other words, it can not simultaneously increase strength and impact toughness.
As organic-inorganic hybrid nanocomposite possesses the strength and toughness of the organic
object and the rigidity, thermal stability and function of the inorganic object, indicating synergistic
enhancement of the hybrid compounds in nanoscale, it becomes the fourth generation of new
materials which are after one-component materials, composite materials and functional gradient
materials. It has become a hot research topic in the field of materials science.
In this context, this study attempts to use functional monomers with flexible chain unit and
polymerizable C=C double bond as the organic object, and tetraethoxysilane (TEOS) as the inorganic
agent, and duplicate silane as the coupling agent, to form organic-inorganic hybrid polymer within
the natural cell pores of wood. The purpose is to impart the high strength, high toughness of the
polymer and thermal stability of the inorganism to the untreated wood and accordingly form an
integrated wood .
2. Experiments and materials
2.1. Test materials
Glycidyl methacrylate(GMA) (Shanghai Yuanji Chemical Co. Ltd..) and 2,2-
Azobisisobutyronitrile(AIBN) (Shanghai Reagent 4 Factory) are analytical Reagents (AR).
Poly(ethylene glycol) dimethacrylate (PEG200DMA) (Yantai Yunkai Chemical Co., Ltd..) is
chemically purified (CP) agent. Tetraethoxysilane (TEOS)( Tianjin Komio Chemical Reagent
Development Cente) is analytical reagent(AR). γ-( methacryloxypropyl) trimethoxy silane (KH570)
(Nanjing Shuguang Chemical Group Co., Ltd..) is chemically purified agent. Laboratory chemical
reagents such as hydrochloric acid(38wt), tetrahydrofuran, acetone, anhydrous ethanol, deionized
water, are analytical reagent. All these chemical reagents can be used directly.
The wood species is Populus Ussuriensis Komarov, which was collected from Heilongjiang
Maoershan Forest Plant. The size of the wood plates is 25×300×2000 mm
3
(radial (R)×tangential (T)
×longitudinal (L)). After three-month adjustment at room temperature, the average moisture content
of the wood plate reached 10.4 wt%. Then the specimens are dried at 105
o
C for 24h to form an
average density of 0.35 g/cm
3
, and further stored for use afterward.
The experimental equipments and instruments include impregnation tank (home-made with size
of 300mm × 600mm (inner diameter × high) and maximum pressure of 1.7 MPa), vacuum oven
(DZF-6050 purchased from Shanghai Precision Instrument Instrument Co., Ltd.), WL-1 Particle Ball
Mill (Tianjin Computer Room Equipment Factory), Atomic-force microscopy (AFM, NaioAFM,
Nanosurf AG, Liestal, Switzerland) with tapping mode, QUANTA2000 Environmental Scanning
Electron Microscope (ESEM) (FEI Company), Magna IR 560 Fourier Transform Infrared
Spectroscopy (FTIR) (Nicolet Company in US), D/max2200 X-ray diffraction instrument (Rigaku
Corp., Tokyo, Japan) (XRD), and Thermogravimetric Analysis (TG/DTG, Q5000IR, TA Instrument,
New Castle, Delaware).
Property Improvement of Wood by Organic-inorganic Hybrid Polymer
631