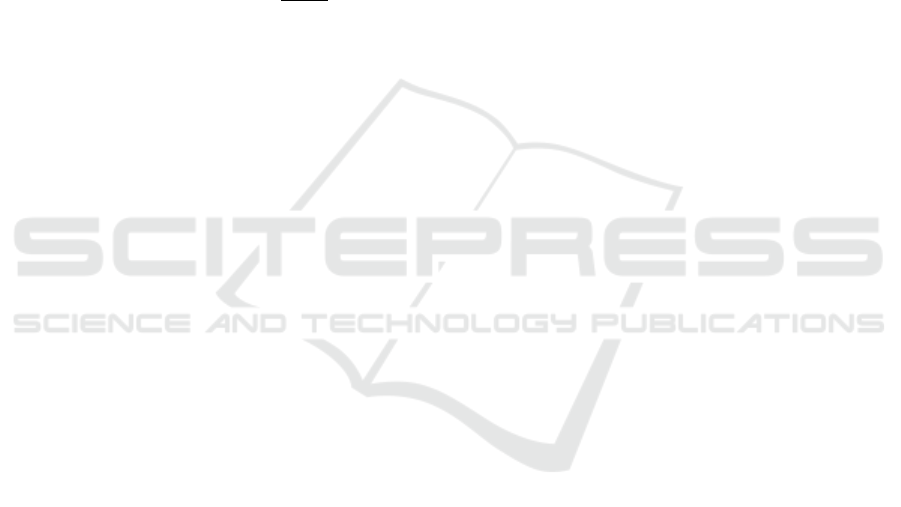
where xi, X
i
, X
0
, and ΔX
i
represent coded value, real value, real value at center point, and step
change value, respectively. Results were analyzed through ANOVA. These variables were selected
based on results of preliminary experimentation. CO
2
concentration and light intensity were used to
prepare 13 experimental formulations.
3. Results and discussion
3.1. Effect of initial NH4-N concentration
Figure 1.a depicts the curves of NH
4
-N removal rate at different initial NH
4
-N concentrations for
seven days of batch operation. NH
4
-N was removed above 50% from the media when initial
concentration was below 79.8 mg/L. Increasing initial NH
4
-N concentration resulted in decreased
NH
4
-N removal rate (23.2%) at NH
4
-N concentration of 332 mg/L. These results indicate that
C. vulgaris can effectively decrease NH
4
-N concentrations to below 111 mg/L. Initial substrate
removal rate is calculated in Eq. (4).
(4)
where P
i
, q
0
, and q
t
represent substrate removal rate, initial NH
4
-N concentration, and corresponding
NH
4
-N concentration, respectively at t
t
, which is the time at which biomass DW did not change
significantly. Specific rate of substrate removal (P
bi
) is determined in Eq. (5).
P
bi
= P
i
/DW
0
(5)
As depicted in Figure 1.b, specific rate of substrate removal decreased with increasing initial NH
4
-
N concentration. Maximum and minimum specific removal rates reached 11.21 and 3.65 d
-1
,
respectively, when specific rate of substrate removal and initial NH
4
-N concentration showed a linear
relationship
3.2. BBD statistical analysis on effects of CO
2
concentration, light intensity, and phosphorus
concentration on nitrogen removal rate
A BBD experiment was performed using Design-Expert 8.0.6, in which removal rate corresponds to
a specified range for optimal culture condition, to investigate three factors (CO
2
, light intensity, and
phosphorus concentration). As suggested by Statistical Model Fit Summary, a mathematical
regression model was fitted as the best model, as indicated by Eq. (6):
Removal rate = 32.93-2.57 × A−0.40 × B+1.78 × C+2.25 × A × B-2.81 × A × C-5.35 × B × C-7.76×
A
2
-2.79 × B
2
-1.12 × C
2
(6)
ANOVA results showed that among the parameters, light intensity presents the highest F-value of
1.34 and lowest p-value of 0.2854, revealing that this factor more significantly influence NH
4
-N
removal in model diesel. Parameters could not be analyzed independently because of interaction
effects between variables. Figure 2 provides the contour plots of NH
4
-N removal conversion; the
plots indicate interaction effects using a regression equation. When phosphorus concentration
remained unchanged, light intensity and CO
2
showed slightly remarkable interaction effect on the
removal rate of NH
4
-N. When CO
2
concentration decreased (6%), NH
4
-N removal rate increased
with increasing light intensity. When CO
2
concentration increased from 6% to 10%, removal rate and
light intensity increased with direct proportions. The same situation was also noted for light intensity.
NH
4
-N removal rate was inversely and directly proportional to low and high light intensity and CO
2
concentrations, respectively. Raised surfaces were detected at CO
2
concentration ranges and light
intensities of 2% to 4% and 5000–7500 lx and 8% to 10% and 12500–15000 lx, respectively,
indicating that light intensity and CO
2
are essential for photosynthesis. Extremely high or low levels
of these factors can inhibit photosynthesis. Figure 2.b and Figure 2.c respectively show remarkable
Optimization of Culture Conditions for Chlorella Vulgaris Using Corresponding Normal Surface and Determination of Coefficients for
Batch Kinetics of Nitrogen Removal Rate
519