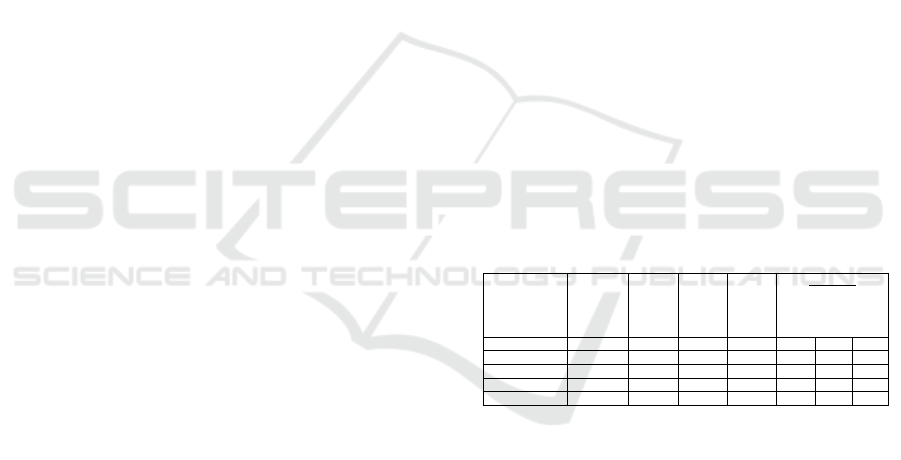
range of pulse frequency: 0-15 MHz; the range of
average export power: 0-500 W. These parameters
are detected by oscillograph (TDS1012B-type, fitted
P6015A-1000X-type high voltage detector and
A621-type current detector, America Tektronix
Company) in real time.
2.2 Analysis Method
The quantitative analysis of DFP is operated by GC
(7890A-type, fitted FPD detector, Agilent
Company). The analysis of active particles is
operated by spectrometer (AvanSpec-2048 type,
fitted fiber probe, Avantes Company).
The plasma reactor and the spectral probe are
placed in the spectral detection chamber, and the
fiber probe is fixed in the plasma reactor quartz
medium tube. The analysis process is as follows.
Firstiy, open the AvanSpec-2048 spectrometer and
perform parameter setting (integration time, etc.).
Secondly, the pure gas spectrum is detected.
Thirdly, the air discharge spectrum is detected, thus
the type and amount of the active particles are
determined. Finally, spectral detection was
performed before and after the air plasma
degradation reaction.
3 RESULTS AND DISCUSSION
3.1 Analysis of Spectra
To study the spectral changes of active particles
during plasma degradation of DFP, this study
intends to determine the characteristic peaks of
several common active particles such as
[O],[O
+
],[O
2
+
] by the emission spectrum
experiments and literature review of pure gas (N
2
,
O
2
, Ar) and air environment. Due to the plasma
reaction, particle level structure and interference
between particles, the paper believes that the
selection of characteristic peaks of active particles
should meet the following principles:
Firstly, the peaks are stably present in the plasma
of different background gases, which is the basis for
determining the characteristic peaks of the active
particles; Secondly, the intensity of the peak is
sensitive to the discharge power. The amount of
active particles is closely related to the input energy.
The active particles may have dozens of lines. Some
of the lines may vary with energy, and the intensity
changes are not obvious. Therefore, it is necessary
to select those spectral lines that are more sensitive
to energy (power) as characteristic peaks; Thirdly,
the spectral intensity is high and easy to distinguish;
Fourthly, the spectrum peak is away from
characteristic peaks of other active particles.
Overlapping peaks may exist because of many
spectrum lines from different active particles. If the
location of two peaks is nearer, they may overlap
affected by resolution ratio.
Take [O] for example, according to spectrum
data comparison between literatures (Xie, 2008;
Zhang et al., 2002) and this research, approximative
wavelength peaks were searched. The principles
were also used to treat these peaks.
(1) The spectrum character of [O] in discharging
condition of pure oxygen
The spectrum of pure oxygen (gas flow 100 L/h)
with the DBD plasma was detected in three different
power of 74.4 W, 119.2 W and 154.8 W. comparing
with literature, 15 rather apparent peaks of [O]
(394.75 nm,395.38 nm,543.50 nm,700.19 nm,700.77
nm,700.82 nm,725.17 nm,725.39 nm,747.64
nm,777.28 nm,778.11 nm,795.63 nm,822.53
nm,844.73 nm,845.08 nm) were discovered. 5
spectrum peaks (543.50 nm,700.77 nm,700.82
nm,822.53 nm,844.73 nm) that its intensity is
stronger with the power rising were selected by
comparing the spectrum intensity of different power,
as is shown in Table 1.
Table 1: The spectrum intensity of [O] in discharging
condition of pure oxygen with different power.
1.0:1.6:2.1
The spectrum
intensity ratio under
condition of three
power
Note: Regarding the spectra intensity value of the lowest power
as basis, and divided by all spectra intensity value of different power
of each wavelength. This is the calculation method of spectra intensity
ratio. Take 543.5 nm, for example, 78.4/78.4=1.0,101.6/78.4=1.3,
135.8/78.4=1.7. The style of level is low level-high level.
The 5 peaks are sensitive to the power and
stronger with the power rising. The energy gap of
excited state and ground state is different. After
eradiated transition, peaks of different wavelength
are emitted. Spectrum intensity of 700.77 nm is
insensitive to power, while the intensity of other
four peaks is stronger with the power rising. The
energy requirement of excited state is different with
different energy levels. Jump to the lower level
5
S
0
,
3
S
0
, the intensity ratio of 543.50 nm and 844.73
nm peaks is 1.0:1.3:1.7 and 1.0:1.4:3.0 when the
power ratio is 1.0:1.6:2.1. So the intensity change is
obvious. The reason for the difference of spectrum
intensity ratio of different peaks is the quantum
MEEP 2018 - The Second International Conference on Materials Chemistry and Environmental Protection
60