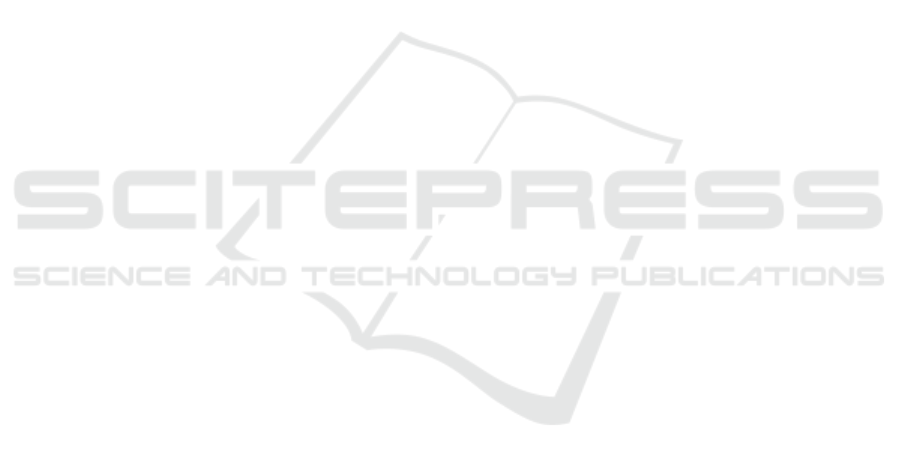
4 CONCLUSIONS
In summary, CuO nanoflakes at gas-liquid interface
was fabricated by a facile CBD method. The product
was composed of uniform and rhombus nanoflakes
with high crystalline. The CuO nanoflakes exhibited
excellent photocatalytic activity with the addition of
H
2
O
2
and the corresponding photodegradation rate
was 85.9%. The results indicated that fabricating
materials on gas-liquid interface via chemical bath
deposition was a promising method.
ACKNOWLEDGEMENTS
This work was co-supported by the National Natural
Science Foundation of China (No. 21571068), the
Natural Science Foundation of Guangdong Province
(No. 2015A030313387) and the Science and
Technology Program of Guangzhou (No.
201607010301).
REFERENCES
Ahmadi, M., Siadati, M.H., 2018. Synthesis, mechanical
properties and wear behaviour of hybrid
Al/(TiO
2
+CuO) nanocomposites. Journal of Alloys and
Compounds. 769, 713-724.
Andana, T., Piumetti, M., Bensaid, S., Veyre, L. et al.,
2017. CuO nanoparticles supported by ceria for
NOx-assisted soot oxidation: insight into catalytic
activity and sintering. Applied Catalysis B:
Environmental. 216, 41-58.
Borders, T., Wang, L., Fushimi, R., Redekop, E. et al., 2018,
Pulse response analysis using the Y-produce: A data
science approach. Chemical Engineering Journal. 192,
46-60.
Dong, C.J., Xing, X.X., Chen, N., Liu, X., Wang, Y.D.,
2016, Biomorphic synthesis of hollow CuO fibers for
low-ppm-level n-propanol detection via a facile
solution combustion method. Sensor. Actuat. B-Chem.
230, 1-8.
Du, X.D.,Zhang, Y.Q.,Si, F., Yao, C.H., Du, M.M. et al.,
2019, Persulfate non-radical activation by nano-CuO
for efficient removal of chlorinated organic
compounds: Reduced graphene oxide-assisted and
CuO (0 0 1) facet-dependent. Chemical Engineering
Journal.356, 178-189.
Hoshyarmanesh, H., Ghodsi, M., Park, H.H., 2016.
Electrical properties of UV-irradiated thick film
piezo-sensors on superalloy IN718 using
photochemical metal organic deposition. Thin Soild
Films. 616, 673-679.
Hou, L., Zhang, C.M., Li, L., Du, C., Li, X.K. et al., 2018.
CO gas sensors based on p-type CuO nanotubes and
CuO nanocubes: Morphology and surface structure
effects on the sensing performance. Talanta. 188,
41-49.
Hsieh, K., Wang, H. J., Locke, B.R., 2016. Analysis of a
gas-liquid film plasma reactor for organic compound
oxidation. Journal of Hazardous Materials.317,
188-197.
Huang, X.M., Ding, J., Zhong, Q., 2015. Catalytic
decomposition of H
2
O
2
over Fe-based catalysts for
simultaneous removal of NO
X
and SO
2.
Applied
Surface Science. 326, 66-72.
Li, D., Fang, M.J., Jiang, C.L. et al., 2018. Size-controlled
synthesis of hierarchical bismuth selenide nanoflowers
and their photocatalytic performance in the presence
of H
2
O
2
. Journal of nanoparticle research. 20.
Long, J., Yang, Z.H., Zeng, X., Huang, J.H., 2017.
Self-assembly of exfoliated layered double hydroxide
and graphene nanosheets for electrochemical energy
storage in zinc/nickel secondary batteries. Journal of
Powder Source.359, 111-118.
Ma, X.Y., Xiang, Q.J., Liao, Y.L., Wen, T.L., Zhang, H.W.,
2018. Visible-light-driven CdSe quantum
dots/graphene/TiO
2
nanosheets composite with
excellent photocatalytic activity for E. coli disinfection
and organic pollutant degradation. Applied Surface
Science. 457, 846-855.
Sudrajat, H., Hartuti, S., 2018. Structural properties and
catalytic activity of a novel ternary CuO/gC
3
N
4
/ Bi
2
O
3
photocatalyst. Journal of Colloid and Interface
Science. 524, 227-235.
Terasako, T., Murakami, T., Hyodou, A., Shirakata, S.,
2015. Structural and electrical properties of CuO films
and n-ZnO/p-CuO heterojunctions prepared by
chemical bath deposition based technique. Solar
Energy Materials and Solar Cells.132, 74-79.
Wang, Q.L., Li, H.Y., Yang, J. H., Sun, Q., Li, Q.Y., Yang,
J.J., 2016. Iron phthalocyanine-graphene
donor-acceptor hybrids for visible-light-assisted
degradation of phenol in the presence of H
2
O
2
. Applied
Catalysis B: Environmental. 192, 182-192.
Yang, J., Cho, M., Lee, Y.K., 2016. Synthesis of
hierarchical Ni(OH)
2
hollow nanorod via chemical bath
deposition and its glucose sensing performance.
Sensor. Actuat. B-Chem. 222, 674-681.
Zhang, G.Q., Li, Z., Wang, X., Zheng, H.Y., Hao, Z.Q.,
Wang, J.J., 2016. Influence of surface oxygenated
groups on the formation of active Cu species and the
catalytic activity of Cu/AC catalyst for the synthesis of
dimethyl carbonate. Applied Surface Science.390,
68-77.
Zhang, N., Zhang, Y.H., Xu, Y.J., 2012. Recent progress on
graphene-based photocatalysts: current status and
future perspectives. Nanoscale. 4, 5792-5813.
Fabrication of CuO Nanoflakes at Gas-liquid Interface Via Chemical Bath Deposition with High Photocatalytic Activity
211