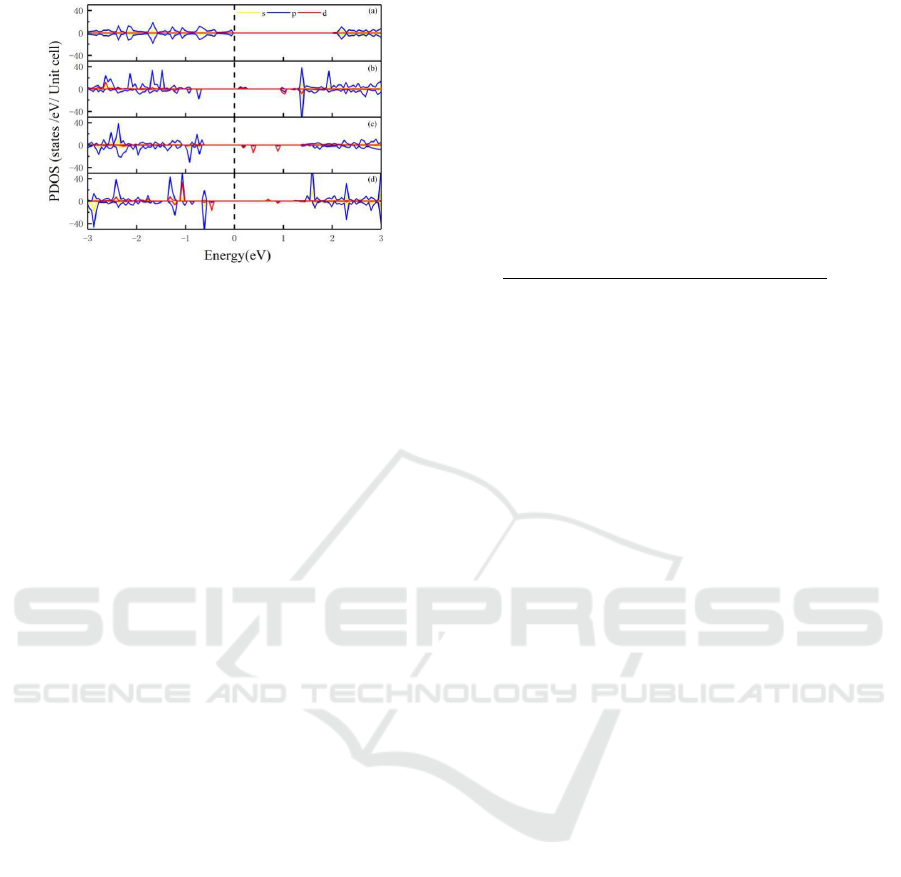
Figure 4: PDOS illustration: (a) ZPNR, (b) ZFe01, (c)
ZFe02, (d) ZFe03.
4 CONCLUSIONS
In summary, based on the density functional
calculations, we have explored the structure and
magnetic properties of doped black phosphorene
nanoribbon. The result show that doped nanoribbon
had lattice distortion but the structure is stable. The
substitution of Fe for P induce magnetic moment
and this moment is localized. The coupling between
the Fe atoms and adjacent P atoms can be either
ferromagnetic or anti-ferromagnetic coupling,
relavent to the magnetic value of Fe atom, which is
required further research.
ACKNOWLEDGEMENTS
This research was supported by Basic Science
Research Program through the Natural Science
Foundation of China (No. 11804169 and 11747029)
and by the Research initiation funds of Nanjing
University of Posts and Telecommunications No.
NY216029. In addition, I appreciate the help from
Dr. Huang Xin for useful discussion.
REFERENCES
Cao, C. et al., 2018. “Transition metal adatom and dimer
adsorbed on graphene: Induced magnetization and
electronic structures”, Physical Review B, Vol. 81 No.
20, pp. 2498-2502.
Dudarev, S. L. et al., 1998. “Electron-Energy-Loss
Spectra and the Structural Stabilityof Nickel Oxide:
An LSDA+U Study”, Physical Review B, Vol. 57 No.
3, pp. 1505-1509.
Kresse, G. and Furthmüller, J., 1996. “Efficient iterative
schemes for ab initio total-energy calculations using a
plane-wave basis set”, Physical Review B, Vol. 54 No.
16, pp. 11169-11186.
Li, L. et al., 2014. “Black phosphorus field-effect
transistors”, Nature Nanotechnology, Vol. 9 No. 5, pp.
372-377.
Liu, S. X. et al., 2017. “Graphene/phosphorene nano-
heterojunction: facile synthesis, nonlinear optics, and
ultrafast photonics applications with enhanced
performance”, Photonics Research, Vol. 5 No. 6, pp.
662- 668.
Luan, Z. et al., 2017. “First-principles study on electronic
structures and magnetic properties of Eudoped
phosphorene”, Superlattices and Microstructures.
http://dx.doi.org/10.1016/j.spmi.2017.07.039
Peng,X. et al., 2014. “Edge effects on the electronic
properties of phosphorene nanoribbons”, Journal of
Applied Physics, Vol. 116 No. 14, p. 144301
Perdew, J. P. et al., 1996a. “Generalized Gradient
Approximation Made Simple”, Physical Review
Letters, Vol. 77 No. 18, pp. 3865-3868.
Perdew, J. P. et al., 1996b. “Generalized Gradient
Approximation Made Simple”. Physical Review
Letters, Vol. 78 No. 7, p. 3865.
Sharma, D. K. et al., 2018. “Magnetism by embedding 3d
transition metal atoms into germanene”, Journal of
Physics D-Applied Physics, Vol. 51 No. 22.
Son, Y. W. et al., 2006. “Half-metallic graphene
nanoribbons”, Nature, Vol 444 No. 7117, pp. 347-349.
Sun, M. L. et al., 2016. “Magnetism in transition metal-
substituted germanane: A search for room temperature
spintronic devices”, Journal of Applied Physics, Vol.
119 No. 14, pp. 666-672.
Zhai, C. Y. et al., 2017. “Strain tuning of magnetism in
transition-metal atom doped phosphorene”,
Superlattices and Microstructures, Vol. 101, pp. 49-
56.
Zhou, W. Z. et al., 2017. “Doping effects on the electronic
properties of armchair phosphorene nanoribbons: A
first-principles study”, Physica E: Low-dimensional
Systems and Nanostructures, Vol. 94, pp. 53-58.
Zhu, Z. L. et al., 2014. “Magnetism of zigzag edge
phosphorene nanoribbons”, Applied Physics Letters,
Vol. 10, p. 113105.
Magnetic Properties of Edge Hydrogenated Zigzag Black Phosphorene Nanoribbon by Fe Doping
231