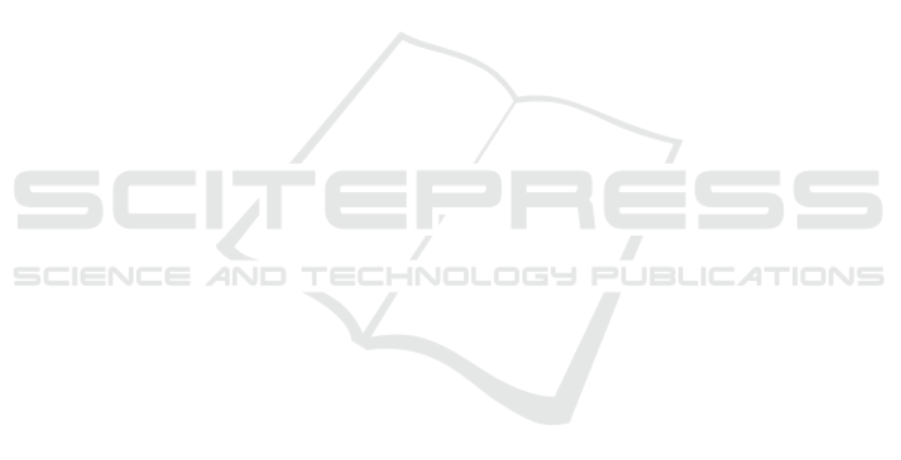
and selection can be effectively inhibited, and
eventually the population tends to become consistent
(Whitlock, 1999). However, when the gene flow
(Nm) between populations is less than one, genetic
differentiation may occur due to the obstruction of
gene flow among sub-populations (Chen, et al.,
2004).
In this experiment, the gene flow (Nm) of each
microsatellite locus ranged from 0.226 to 0.553, with
an average of 0.375, which revealed that the gene
exchange between the two populations of
Reticulitermes aculabialis in Xi'an and Nanjing was
weak or non-existent, resulting in a higher genetic
differentiation among populations.
The reason for the low gene exchange between the
two populations was that the termites could not
migrate over a wide range due to the short and limited
flight time and poor flight ability, which limited the
genetic communication among the populations.
Additionally, urban areas were densely populated,
and human activities were frequent. Inter-city eco-
tourism and urbanization construction led to
environmental fragmentation, leading to isolated
nesting and breeding of termites, which in turn
affected genetic communication among populations.
ACKNOWLEDGEMENTS
This work was financially supported by scientific
research project in school-level of Xi’an Pei hua
University (PHKT18064).
REFERENCES
Barton, N. H., Etheridge, A. M., 2010. Véber A. A new
model for evolution in a spatial continuum[J]. Electron
J Probab, 15(7): 162-216.
Barton, N., 2001. The role of hybridization in evolution[J].
Molecular Ecology, 10(3): 551-568.
Chand, R. R., Jokhan, A. D., Charan, H. et al., 2018. Threats
posed by Asian subterranean termites in the Fiji Islands
and their potential controls: a review[J]. New Zealand
Plant Protection, 71: 129-139.
Chen, J., Dong, C., Sun, D. et al., 2004. Genetic variation
analysis of chum salmon populations in Heilongjiang
river based on microsatellite markers[J]. Acta
Hydrobiologica Sinica, 28(6):607-612.
Dronnet, S., Bagneres, A. G., Juba, T. R. et al., 2004.
Polymorphic microsatellite loci in the European
subterranean termite, Reticulitermes santonensis
Feytaud[J]. Molecular Ecology Notes, 4(1): 127-129.
Jsc, S., Ecl, C., Shu, H. et al., 1997. An evaluation of the
utility of SSR loci as molecular markers in maize (Zea
mays L.): comparisons with data from RFLPS and
pedigree[J]. Theoretical and Applied Genetics, 95(1):
163-173.
Lewontin, R. C., 1995. The Apportionment of Human
Diversity[J]. Bmc Evolutionary Biology, ,6: 381-398.
Li, G. X., Rong, D. Z., and Yang, B., 2010. "Introduction
to termite research in China." Journal of Applied
Entomology 117.1-5(2010):360-369.
Nei, M., 1973. Analysis of gene diversity in subdivided
populations[J]. Proceedings of the National Academy
of Sciences of the United States of America, 70(12):
3321.
Nei, M., Takezaki, N., 1996. The root of the phylogenetic
tree of human populations[J]. Molecular Biology &
Evolution, 13(1): 170-177.
Peakall, R., Smouse, P. E., 2012. GenAlEx 6.5[J].
Bioinformatics, 28(19): 2537-2539.
Rod, P., Petere, S., 2006. GENALEX 6: genetic analysis in
Excel. Population genetic software for teaching and
research[J]. Molecular Ecology Notes, 6(1): 288-295.
Rust, M. K., Su, N. Y., 2012. Managing Social Insects of
Urban Importance[J]. Annual Review of Entomology,
57(57):355.
Vargo, E. L., 2000. Polymorphism at trinucleotide
microsatellite loci in the subterranean termite
Reticulitermes flavipes[J]. Molecular Ecology, 9(6):
817–820.
Vargo, E. L., 2003. Hierarchical analysis of colony and
population genetic structure of the eastern subterranean
termite, Reticulitermes flavipes, using two classes of
molecular markers[J]. Evolution, 57(12): 2805-2818.
Vargo, E. L., Henderson, G., 2000. Identification of
polymorphic microsatellite loci in the Formosan
subterranean termite Coptotermes formosanus
Shiraki[J]. Molecular Ecology, 9(11): 1935-1938.
Wang, H., Chang, H., Xu, W. et al., 2004. Genetic analysis
of microsatellite DNA markers in domestic quail and
wild japanese quail populations[J]. Chinese Journal of
Animal & Veterinary Sciences, 20(8):1-6.
Whitlock, M. C., 1999. Neutral additive genetic variance in
a metapopulation[J]. Genetical Research, 74(3):215-
221.
Whitlock, M. C., Mccauley, D. E., 2010. Indirect measures
of gene flow and migration: FST≠1/(4Nm+1)[J].
Heredity, 82(82): 117-125.
Wright, S., 1974. Evolution in Mendelian populations[M].
New directions in police-community relations.
Rinehart Press, 1974: 241-295.
Wright, S., 1978. Evolution and the genetics of populations:
a treatise in four volumes: Vol. 4: variability within and
among natural populations[M]. University of Chicago
Press.
Xing, L., Cui, H., and Cheng, J., 1998. Foraging
populations and territories of Reticulitermes aculabialis
Tsai et Hwang (Isoptera: Rhinotermitidae) in urban
environment[J]. Journal of Zhejiang Agricultural
University.
Zhong, J. H., Liu, L. L., 2002. Termite fauna in China and
their economic importance.[J]. Sociobiology, 40:25-32.
Study on the Genetic Diversity of Reticulitermes Aculabialis
259