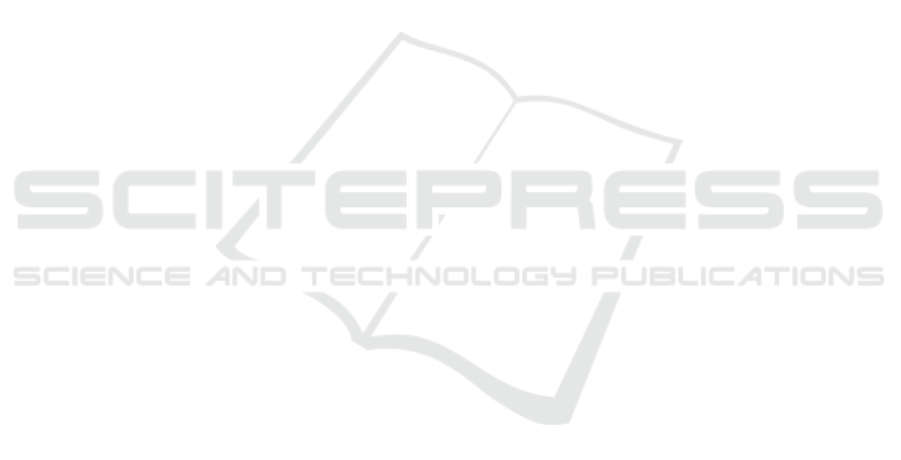
anisotropic grains which were not detected by XRF
are probably sulphides. Spherical particles which
appear dark in both transmitted and reflected light
correspond to wüstite. Brucite forms the fine eutectic
structure, probably with wüstite needles.
Structures like the ones in Figure 3b and 5 are too
fine to be analised correctly by EDX in SEM. Also,
different phases will only show grey tone contrast in
SEM. XRD cannot detect phases which are present in
small amounts, nor amorphous components, which
are readily identified in OM. As EDX cannot quantify
oxygen, discrimination between different metal
oxides and hydroxides is often not possible, while it
is fairly straightforward in a petrographic
microscope.
For the present materials, the limited resolution of
OM poses no problem. One drawback is that most
reference works on OM refer to geological materials.
Reference to man-made waste materials is not
available. Additional SEM/EDX analysis of the thin
slices may help to solve this limitation in future work.
4 CONCLUSIONS
Selection of slag materials for specific applications of
environmental engineering requires the identification
of potentially active components. Petrographic
microscopy is a classical tool used by geologists to
elucidate the mineralogical composition of rocks but
is rarely used outside this speciality and has been
partially substituted by SEM. Here it was shown that
it provides valuable details on the microstructure and
phase distribution in slags, especially when combined
with SEM, EDX and XRD. The technique has proven
particularly useful in the analysis of the oxides and
hydroxides of Fe, Mg and Ca, which play a
fundamental role in contaminant removal.
ACKNOWLEDGEMENTS
This work was sponsored by DGAPA project IV100616.
REFERENCES
Abu-Eishah, S.I., El-Dieb, A.S., Bedir, M.S., 2002.
Performance of concrete mixtures made with electric
arc furnace (EAF) steel slag aggregate produced in the
Arabian Gulf region. Constr. Build. Mater, 34, 249.
Barca, C., Troesch, S., Meyer, D., Drissen, P., Andres, Y.,
Chazarenc, F., 2012. Steel slag filters to upgrade
phosphorus removal in constructed wetlands: two years
of field experiments. Environm. sci. tech., 47, 549.
Chazarenc, F., Kacem, M., Gerente, C., Andres, Y., 2008.
Active filters: a mini-review on the use of industrial
by-products for upgrading phosphorus removal from
treatment wetlands. In Proc. 11th Internat. Conf.
Wetland Systems for Water Pollution Control. IWA.
Dimitrova S.V., Mehanjiev D.R., 2000. Interaction of
blast-furnace slag with heavy metal ions in water
solutions. Water Res. 34, 1957.
Goldstein, J.I., Newbury, D.E., Michael, J.R., Ritchie,
N.W., Scott, J.H.J., Joy, D.C. 2017. Scanning electron
microscopy and X-ray microanalysis. Springer.
Kourounis, S. Tsivilis, S. Tsakiridis P.E., Papadimitriou G.
D., Tsibouki Z., 2007. Properties and hydration of
blended cements with steelmaking slag. Cement Concr.
Res. 37 815.
Maslehuddin, M., Sharif, A.M., Shameem, M., Ibrahim,
M., Barry, M.S., 2003. Comparison of properties of
steel slag and crushed limestone aggregate
concretes. Constr. Build. Mater., 17, 105.
Mercado-Borrayo, B.M., Schouwenaars, R.,
González-Chávez, J.L., Ramírez-Zamora, R.M., 2013.
Multi-analytical assessment of iron and steel slag to
estimate the removal of metalloids from contaminated
water. J. Environm. Sci. Health, A, 48, 887.
Mercado-Borrayo, B.M., González-Chávez, J.L.,
Ramírez-Zamora, R.M., Schouwenaars, R., 2018a.
Valorisation of Metallurgical Slag for the Treatment of
Water Pollution: An Emerging Technology for
Resource Conservation and Re-utilization. J. Sust.
Metall., 4, 50.
Mercado-Borrayo, B.M., Solís-López, M., Schouwenaars,
R., Ramírez-Zamora, R.M., 2018b. Application of
metallurgical slag to treat geothermal wastewater with
high concentrations of arsenic and boron. Int. J.
Environm. Sci. Tech., 1.
Mercado-Borrayo, B.M., Contreras, R., Sánchez, A., Font,
X., Schouwenaars, R., Ramírez-Zamora, R.M., 2018c.
Optimisation of the removal conditions for heavy
metals from water: A comparison between steel furnace
slag and CeO2 nanoparticles. Arabian J. Chem.
Nesse, W.D., 2009. Introduction to Optical Mineralogy.
Oxford university press.
Piatak, N.M., Parsons, M.B., Seal R.R., 2015.
Characteristics and environmental aspects of slag: A
review. App. Geochem. 57 236.
Qasrawi, H., Shalabi, F., Asi, I., 2009. Use of low CaO
unprocessed steel slag in concrete as fine
aggregate. Constr. Build. Mater., 23, 1118.
Schouwenaars, R., Montoya-Bautista, C.V., Isaacs-Páez,
E.D., Solís-López, M., Ramírez-Zamora, R.M., 2017.
Removal of arsenic III and V from laboratory solutions
and contaminated groundwater by metallurgical slag
through anion-induced precipitation. Environm. Sci.
pollut. res. 24, 25034.
Vohla, C., Kõiv, M., Bavor, H. J., Chazarenc, F., Mander,
Ü., 2011. Filter materials for phosphorus removal from
wastewater in treatment wetlands—A review. Ecol.l
Eng., 37, 70.
MEEP 2018 - The Second International Conference on Materials Chemistry and Environmental Protection
300