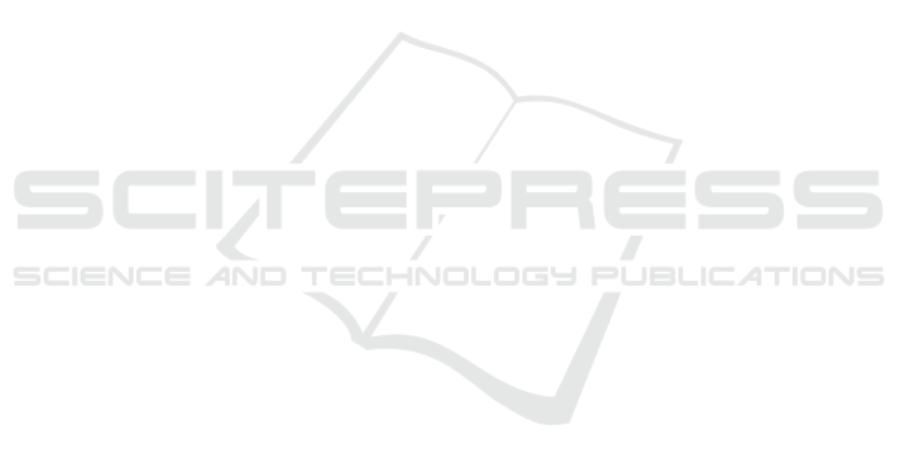
H
2
O
2
diffusion to the interface. Consequently, the
decoloration rate was decreased. On the other hand,
due to a large amount of ·OH radical generated in
proposed process, more OH radicals were scavenged
by H
2
O
2
instead of attacking RhB molecular. The
inhibitory effect of H
2
O
2
could be explained as
follows:
H
2
O
2
+ ·OH → H
2
O + HOO·
In this case, the scavenging effect of H
2
O
2
shows
more influences rather than releasing ·OH radical.
Thus, the decoloration will decrease with the
increasing additive H
2
O
2
.
4 CONCLUSIONS
The present work has shown that RhB can be
effectively removed from aqueous solution by
proposed ultrasound assisted pulse discharge.
Solution electrical conductivity for desirable
discharge type is widely extended. Energy efficiency
of discharge is significantly improved by ultrasound,
especially in higher electrical conductivity of
solution. Additive H
2
O
2
solution shows an inhibitive
effect on RhB decoloration in proposed method.
Additive H
2
O
2
performs as a inhibitor in the
experiments.
ACKNOWLEDGMENTS
This work is supported by China Scholarship
Council.
REFERENCES
Behnajady, M., Modirshahla, N., Tabrizi, S. B. &
Molanee, S. 2008. Ultrasonic degradation of
Rhodamine B in aqueous solution: influence of
operational parameters. Journal of Hazardous
Materials, 152, 381-386.
Bruggeman, P. & Leys, C. 2009. Non-thermal plasmas in
and in contact with liquids. Journal of Physics D:
Applied Physics, 42, 053001.
Fang, Y., Shimizu, S., Yamamoto, T. & Komarov, S.
2018a. Generation of OH Radical by Ultrasonic
Irradiation in Batch and Circulatory Reactor. IOP
Conference Series: Earth and Environmental Science,
120, 012019.
Fang, Y., Yamamoto, T. & Komarov, S. 2018b. Cavitation
and acoustic streaming generated by different
sonotrode tips. Ultrasonics Sonochemistry, 48, 79-87.
Gershman, S., Mozgina, O., Belkind, A., Becker, K. &
Kunhardt, E. 2007. Pulsed electrical discharge in
bubbled water. Contributions to Plasma Physics, 47,
19-25.
Komarov, S., Oda, K., Ishiwata, Y. & Dezhkunov, N.
2013. Characterization of acoustic cavitation in water
and molten aluminum alloy. Ultrason Sonochem, 20,
754-61.
Lee, H., Park, S. H., Park, Y.-K., Kim, B. H., Kim, S.-J. &
Jung, S.-C. 2013. Rapid destruction of the rhodamine
B using TiO 2 photocatalyst in the liquid phase
plasma. Chemistry Central Journal, 7, 156.
Liang, J., Komarov, S., Hayashi, N. & Kasai, E. 2007.
Improvement in sonochemical degradation of
4-chlorophenol by combined use of Fenton-like
reagents. Ultrasonics Sonochemistry, 14, 201-207.
Malik, M. A. 2010. Water purification by plasmas: Which
reactors are most energy efficient? Plasma Chemistry
and Plasma Processing, 30, 21-31.
Medodovic, S. & Locke, B. 2009. Primary chemical
reactions in pulsed electrical discharge channels in
water. Journal of Physics D: Applied Physics, 42,
049801.
Mehrdad, A. & Hashemzadeh, R. 2010. Ultrasonic
degradation of Rhodamine B in the presence of
hydrogen peroxide and some metal oxide. Ultrasonics
sonochemistry, 17, 168-172.
Mehrdad, A., Massoumi, B. & Hashemzadeh, R. 2011.
Kinetic study of degradation of Rhodamine B in the
presence of hydrogen peroxide and some metal oxide.
Chemical engineering journal, 168, 1073-1078.
Merouani, S., Hamdaoui, O., Saoudi, F. & Chiha, M.
2010. Sonochemical degradation of Rhodamine B in
aqueous phase: effects of additives. Chemical
Engineering Journal, 158, 550-557.
Nakagawa, Y., Mitamura, S., Fujiwara, Y. & Nishitani, T.
2003. Decolorization of rhodamine B in water by
pulsed high-voltage gas discharge. Japanese journal of
applied physics, 42, 1422.
Siddique, M., Farooq, R. & Price, G. J. 2014. Synergistic
effects of combining ultrasound with the Fenton
process in the degradation of Reactive Blue 19.
Ultrasonics sonochemistry, 21, 1206-1212.
Sugiarto, A. T., Ito, S., Ohshima, T., Sato, M. & Skalny, J.
D. 2003. Oxidative decoloration of dyes by pulsed
discharge plasma in water. Journal of Electrostatics,
58, 135-145.
Sugiarto, A. T. & Sato, M. 2001. Pulsed plasma
processing of organic compounds in aqueous solution.
Thin solid films, 386, 295-299.
Šunka, P. 2001. Pulse electrical discharges in water and
their applications. Physics of plasmas, 8, 2587-2594.
Van De Moortel, N., Van Den Broeck, R., Degrève, J. &
Dewil, R. 2017. Comparing glow discharge plasma
and ultrasound treatment for improving aerobic
respiration of activated sludge. Water research, 122,
207-215.
Yamabe, C., Takeshita, F., Miichi, T., Hayashi, N. &
Ihara, S. 2005. Water treatment using discharge on the
surface of a bubble in water. Plasma Processes and
Polymers, 2, 246-251.
MEEP 2018 - The Second International Conference on Materials Chemistry and Environmental Protection
310