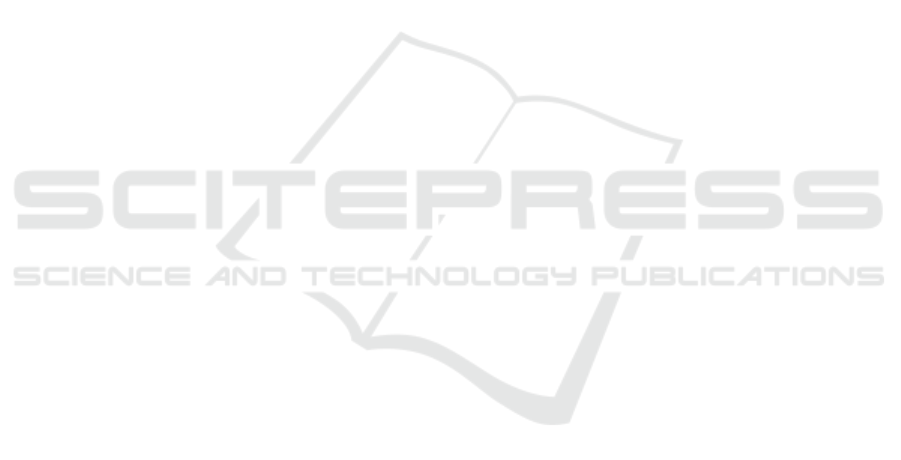
ACKNOWLEDGEMENTS
This research was funded by Directorate of
Research and Community Services, Ministry of
Research, Technology and Higher Education, The
Republic of Indonesia under PUPT research scheme.
The first author would like to thank for the support
provided by the Ministry through The Master’s
Degree Program Leading to Doctoral Degree for
Excellent Bachelor Graduates (PMDSU).
REFERENCES
Abdullah, K., Zubaydi , A. & Budipriyanto, A., 2017.
Development of Sandwich Panel with Core from
Clamshell Powder for Ship Structure. International
Conference on Marine Technology. Surabaya.
Abdullah, K., Zubaydi, A. & Budipriyanto, A., 2018.
Development and Dynamic Analysis of Sandwich
Panels which Core Material Made from Clamshell in
Ship Construction. Department of Naval Architecture,
Institut Teknologi Sepuluh Nopember. Surabaya.
ASTM C 393, 2016. Standard Test Method for Core Shear
Properties of Sandwich Constructions by Beam
Flexure. West Conshohocken. ASTM International.
USA.
Belouettar, S., Abbadi, A., Azari, Z. & Belouet, R., 2009.
Experimental Investigation of Static and Fatigue
Behaviour of Composites Honeycomb Materials Using
Four Point Bending Tests. Composite Structures,
Volume 87 No. 3, pp. 265-273.
Brooking, M. & Kennedy, S., 2004. The Performance,
Safety and Production Benefits of SPS Structures for
Double Hull Tankers. Royal Institution of Naval
Architects. London, UK.
Castanié, B., Aminanda, Y., Bouvet, C. & Barr, J. J., 2008.
Core Crush Criterion to Determine the Strength of
Sandwich Composite Structures Subjected to
Compression After Impact. Composite Structures,
Volume 86 No. 1-3, pp. 243-250.
DNV-GL, 2015. Rules For Classification High Speed and
Light Craft. Part 5 Ship Types Chapter 2 Car Ferry ed.
DNVGL. Norway.
DNV-GL, 2016. Steel Sandwich Panel Construction.
DNVGL. Norway.
DNV-GL, 2017. Rules For Classification Ships. Part 3 Hull
Chapter 4 Loads ed. DNVGL. Norway.
Gunnarson, M., 1994. Beneficial Use of Aluminium in Ro-
Ro Equipment. Department of Naval Architecture and
Ocean Engineering, Chalmers University of
Technology. Göteborg.
Hakansson, M., Johnson, E. & Ringsberg, J. W., 2017. Cost
and Weight of Composite Ship Structures: A
Parametric Study Based on Det Norske Veritas Rules.
Journal of Engineering for the Maritime Environment,
pp. 1-20.
Hanson, K., 2000. Lifting Tires in Aluminum for Vehicle
Transport. Institutionen För Byggkonstruktion,
Kungliga Tekniska Högskolan. Stockholm.
Hassan, , S. B., Aigbodion, V. S. & Patrick, S. N., 2012.
Particulate Composite Development of
Polyester/Eggshell. Tribology in Industry, Volume 34,
pp. 217-225.
Kortenoeven, J., Boon, B. & De Bruijn, A., 2008.
Application of Sandwich Panels in Design and Building
of Dredging Ships. Journal of Ship Production, pp.
125-134.
Llyod’s Register, 2015. Provisional Rules for the
Application of Sandwich Panel Construction to Ship
Structure. Llyod’s Register.London.
Mamalis, A., Manolakos, D., Ioannidis, M. & Kos, P.,
2002. Axial Collapse of Hybrid Square Sandwich
Composite Tubular Components with Corrugated Core:
Numerical Modelling. Composite Structures, Volume
58, pp. 571-582.
Manshuri, Y. & Amalina, M. A., 2014. Hardness and
Compressive Properties of Calcium Carbonate Derived
from Clam Shell Filled Unsaturated Polyester
Composites. Materials Research Innovations, Volume
18, pp. 291-294.
Momčilović, N. & Motok, M., 2009. Estimation of Ship
Lightweight Reduction by Means of Application of
Sandwich Plate System. FME Transactions, Volume
37, pp. 123-128.
Mula, I. A., Zubaydi, A. & Budipriyanto, , A., 2017.
Properties of Sandwich Panel Core from Eggshell
Powder for Ship Structure,. International Conference
on Marine Technology. Surabaya.
Mula, I. A., Zubaydi, A. & Budipriyanto, A., 2018. Static
and Dynamic Analysis of Sandwich Panels Made of
Core Material from Eggshell in Flat Plate
Construction, Department of Naval Architecture,
Institut Teknologi Sepuluh Nopember. Surabaya.
Noury, P., Hayman, B., McGeorge, D. & Weitzenböck, J.,
2005. Lightweight Construction for Advanced
Shipbuilding-Recent Development. s.l.:Det Norske
Veritas.
Ramnath, B. V. et al., 2018. Sea Shells and Natural Fibres
Composites. Materials Today: Proceedings, Volume 5,
p. 1846–1851.
Reis, E. M. & Rizkalla, S. H., 2008. Material
Characteristics of 3-D FRP Sandwich Panels.
Construction and Building Materials, Volume 22 No.
6, pp. 1009-1018.
Ringsberg, J., 2015.. Steel or Composite Car Deck
Structure - A Comparison Analysis of Weight, Strength
and Cost. Analysis and Design of Marine Structures,
pp. 647-658.
SAND.CORe, 2013. Best Practice Guide for Sandwich
Structures in Marine Applications. s.l.:New Rail.
Sujiatanti, S., Zubaydi, A. & Budipriyanto, A., 2018. Finite
Element Analysis of Ship Deck Sandwich Panel.
Applied Mechanics and Materials, Volume 874, pp.
134-139.
Thelandersson, S., 1987. Analysis of Thin-Walled Elastic
Beams. Lund University. Sweden.
Comparative Study on Ferry Ro-Ro’s Car Deck Structural Strength by Means of Application of Sandwich Materials
95