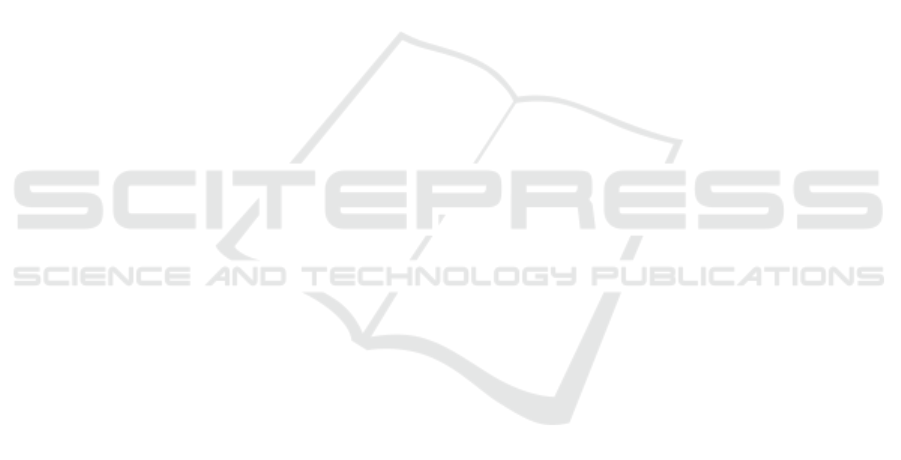
the flight occurs. Repetition of terrain textures and
structures enables stable behaviour and does not
require additional attention of pigeons. Any changes
or additional information – changes in weather
conditions or light, or the appearance of a predator –
may result in changes in the pigeons’ arrangement in
the flock and trajectories. In contrast to individual
birds, the flock perceives the terrain jointly and in
coordination and responds to changes in terrain
cooperatively – with changes not only in trajectories
but also in the flock structure itself.
During a flight over heterogeneous terrain, a
great amount of diverse information is observed.
This information can be generalized and become
typical. Sets of data are extracted from it and can be
applied even when the birds have become
accustomed to operating in other situations.
Accordingly, the responses to similar sets of data
will be alike. This promotes both fast and consistent
responses during flight over similar terrain and
adaptation during flight over different terrain. When
current situation change, the sets of reference points
and regions also change.
Generally, pigeons prefer to fly over already
familiar terrain, gradually extending the survey
areas. But even well-known territories can change –
at different times of day and seasons of the year –
and therefore flock navigational strategies can
change completely, and the flock can even fly away
from previously interesting terrain.
REFERENCES
Ballerini, M. et al. (2008) ‘Interaction ruling animal
collective behavior depends on topological rather than
metric distance: Evidence from a field study’,
Proceedings of the National Academy of Sciences,
105(4), pp. 1232–1237.
Chen, D. et al. (2017) ‘Anisotropic interaction rules in
circular motions of pigeon flocks: An empirical study
based on sparse Bayesian learning’, Physical Review
E, 96(2), p. 022411.
Dell’Ariccia, G. et al. (2008) ‘Flock flying improves
pigeons’ homing: GPS track analysis of individual
flyers versus small groups’, Animal Behaviour.
Academic Press, 76(4), pp. 1165–1172.
Guilford, T. and Biro, D. (2014) ‘Route following and the
pigeon’s familiar area map’, Journal of Experimental
Biology, 217(2), pp. 169–179.
Jolles, J. W. et al. (2017) ‘Consistent Individual
Differences Drive Collective Behavior and Group
Functioning of Schooling Fish’, Current Biology.
Elsevier Ltd., 27(18), p. 2862–2868.e7.
Mann, R. et al. (2011) ‘Objectively identifying landmark
use and predicting flight trajectories of the homing
pigeon using Gaussian processes’, Journal of The
Royal Society Interface, 8(55), pp. 210–219.
Mehlhorn, J. and Rehkaemper, G. (2016) ‘The Influence
of Social Parameters on the Homing Behavior of
Pigeons’, PLOS ONE. Edited by V. P. Bingman,
11(11), p. e0166572.
Morse, D. H. (1977) ‘Feeding Behavior and Predator
Avoidance in Heterospecific Groups’, BioScience.
[American Institute of Biological Sciences, Oxford
University Press], 27(5), pp. 332–339.
Nagy, M. et al. (2013) ‘Context-dependent hierarchies in
pigeons’, Proceedings of the National Academy of
Sciences, 110(32), pp. 13049–13054.
Oloo, F. and Wallentin, G. (2017) ‘An Adaptive Agent-
Based Model of Homing Pigeons: A Genetic
Algorithm Approach’, ISPRS International Journal of
Geo-Information, 6(1), p. 27.
Pearce, D. J. G. et al. (2014) ‘Role of projection in the
control of bird flocks’, Proceedings of the National
Academy of Sciences, 111(29), pp. 10422–10426.
Reynolds, C. W. (1987) ‘Flocks, herds and schools: A
distributed behavioral model’, ACM SIGGRAPH
Computer Graphics, 21(4), pp. 25–34.
Santos, C. D. et al. (2014) ‘Temporal and contextual
consistency of leadership in homing pigeon flocks’,
PLoS ONE. Edited by G. G. de Polavieja, 9(7), p.
e102771.
Topaz, C. M., Ziegelmeier, L. and Halverson, T. (2015)
‘Topological Data Analysis of Biological Aggregation
Models’, PLOS ONE. Edited by B. Ermentrout, 10(5),
p. e0126383.
Vicsek, T. et al. (1995) ‘Novel Type of Phase Transition
in a System of Self-Driven Particles’, Physical Review
Letters, 75(6), pp. 1226–1229.
Wallentin, G. and Oloo, F. (2016) ‘A model-sensor
framework to predict homing pigeon flights in real
time’, GI_Forum, 1, pp. 41–52.
Watts, I. et al. (2016) ‘Lack of experience-based
stratification in homing pigeon leadership hierarchies’,
Royal Society Open Science, 3(1), p. 150518.
Yomosa, M. et al. (2015) ‘Coordinated Behaviour in
Pigeon Flocks’, PLOS ONE. Edited by C. K.
Hemelrijk, 10(10), p. e0140558.
Flock Patterns When Pigeons Fly over Terrain with Different Properties
341