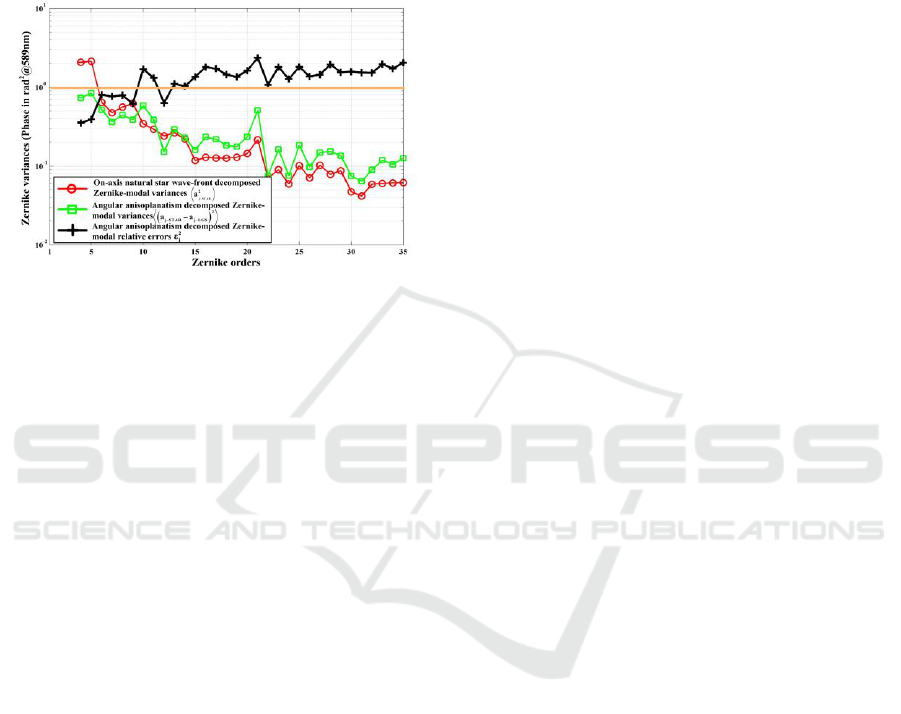
axis sodium LGS, respectively (e.g., the average of
the Zernike-modal correlation <r
j
>=0.70 for j=4~9).
Increasing the Zernike-modal order j means
deteriorating correlation r
j
, the average of the
Zernike-modal correlation <r
j
>=0.46 for j=10~15,
but the average of the Zernike-modal correlation
<r
j
>=0.33 for j=16~20.
Figure 7: The typical experimental result of the angular
anisoplanatism decomposed Zernike-modal relative errors
for the off-axis sodium LGS with 10” angular offsets (Luo
et al., 2018).
The corresponding angular anisoplanatism
decomposed Zernike-modal relative errors for the
off-axis sodium LGS are calculated and illustrated in
Figure 7 (Luo et al., 2018). Resulting from improper
turbulent atmosphere probing with the off-axis
sodium LGS as reference on the outside of the
optical path from the natural star to the telescope
aperture, obvious de-correlations occur between the
majority of Zernike modes of the two types of wave-
front distortions from the on-axis natural star and the
off-axis sodium LGS, and the corresponding angular
anisoplanatism decomposed Zernike-modal relative
errors are bigger than one. This phenomenon is
basically consistent with our previous numerical
simulation work (Luo et al., 2015).
5 CONCLUSIONS
By means of the structural design of the range gating
mechanism accompanied with the synchronized
timing design of the sodium LGS excitation and
collection, the synchronized return-light spot arrays
through turbulent atmosphere from the science
object and the excited sodium LGS with certain
angular offsets can be collected by using our
developed HS wave-front sensor, which provides a
convenient way to experimental measurement of the
angular anisoplanatism for LGS. Further
investigation in this area will be carried out in the
future.
ACKNOWLEDGEMENTS
This work has been supported by the Young
Scientists Fund of the National Natural Science
Foundation of China (Grant No. 61505215).
REFERENCES
Luo, X., Li, X.Y., Hu, S.J., Huang, K., Wang, X.Y., 2018,
Experimental investigation of angular anisoplanatism
for sodium beacon. Acta Physica Sinica, 67 (9),
099501. (In Chinese)
Luo, X., Li, X.Y., Shao, L., Hu, S.J., Huang, K., 2015.
Investigation of anisoplanatic effect in adaptive optics
for atmospheric turbulence correction. In Proceedings
of SPIE, the 20th International Symposium on High-
Power Laser Systems and Applications. SPIE Press.
Foy, R., Labeyrie, A., 1985, Feasibility of adaptive
telescope with laser probe. Astronomy and
Astrophysics, 152 (2), L29-L31.
Fugate, R.Q., Fried, D.L., Ameer, G.A., Boeke, B.R.,
Browne, S.L., Roberts, P.H., Ruane, R.E., Tyler, G.A.,
Wopat, L.M., 1991, Measurement of atmospheric
wavefront distortion using scattered light from a laser
guide star. Nature, 353 (6340), 144-146.
Humphreys, R.A., Primmerman, C.A., Bradley, L.C.,
Herrmann, J., 1991, Atmospheric-turbulence
measurements using synthetic beacon in the
mesospheric sodium layer. Optics Letters, 16 (18),
1367-1369.
Fugate, R.Q., Ellerbroek, B.L., Higgins, C.H., Jelonek, M.
K., Lange, W.J., Slavin, A.C., Wild, W.J., Winker,
D.M., Wynia, J.M., Spinhirne, J.M., Boeke, B.R.,
Ruane, R.E., Moroney, J.F., Oliker, M.D., Swindle,
D.W., Cleis, R.A., 1994, Two generations of laser-
guide-star adaptive-optics experiments at the Starfire
Optical Range. Journal of the Optical Society of
America A, 11 (1), 310-324.
Bonaccini, D., Hackenberg, W., Avila, G., 1998. Laser
guide star facility for the ESO VLT. In Proceedings of
SPIE, Adaptive Optical System Technologies. SPIE
Press.
Joyce, R., Boyer, C., Daggert, L., Ellerbroek, B., Hileman,
E., Hunten, M., Liang, M., 2006. The laser guide star
facility for the Thirty Meter Telescope. In Proceedings
of SPIE, Advances in Adaptive Optics II. SPIE Press.
Molodij, G., Rousset, G., 1997, Angular correlation of
Zernike polynomials for a laser guide star in adaptive
optics. Journal of the Optical Society of America A, 14
(8), 1949-1966.
Sasiela, R.J., 2007. Electromagnetic wave propagation in
turbulence: evaluation and application of Mellin
transforms, SPIE Press. Washington, 2
nd
edition.
PHOTOPTICS 2019 - 7th International Conference on Photonics, Optics and Laser Technology
108