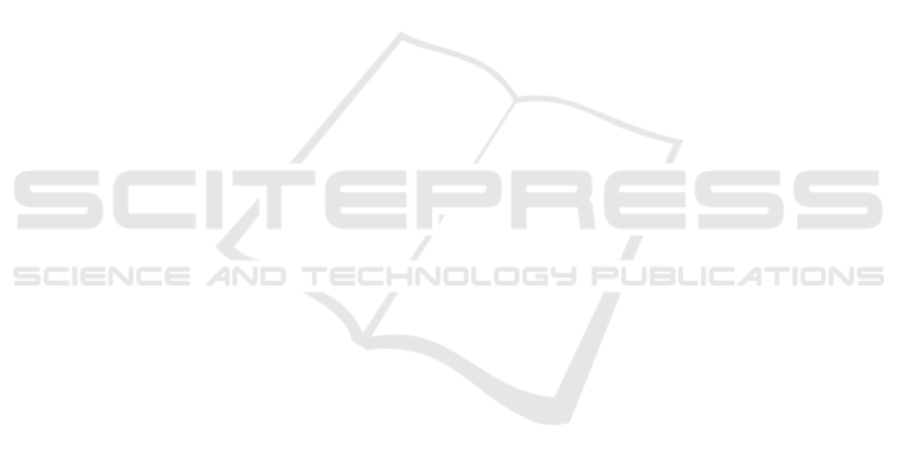
Joan Despí plants, which extract water from the
Llobregat river, the Cardedeu plant, which obtains
water from Ter river, and the Besòs plant, which
treats the underground flows from the aquifer of the
Besòs river. There are also several underground
sources (wells) that can provide water through
pumping stations. Those different water sources
currently offer a flow of around seven m3/s. The
water flow from each source is limited and with
varying prices of water depending on water
treatments and legal extraction canons.
The structure of the Barcelona DWN has two
layers; The upper layer, named transport network, this
layer aims to links the water treatment plants with the
reservoirs distributed all over the city. The lower
layer named distribution network, this layer is
sectored in subnetworks. Each subnetwork links a
tank with each consumer. This application case study
aims to work in the transport network. The control
system of the transport network is also organized in
two layers. The upper layer manages the global
control of the network, establishing the set-points of
the regulatory controllers at the lower layer.
Regulatory controllers are of PID type, while the
supervisory layer controller is of MPC type.
Regulatory controllers hide the network non-linear
behaviour to the supervisory controller. This fact
allows the MPC supervisory controller to use a
control-oriented linear model.
From the whole drinking water network of
Barcelona, described above, this work considers an
aggregated version of this model that is an entirely
representative version of the full network.
Aggregated means that some sectors of the network
are collected in a unique part, hence some tanks are
raised in a single representative tank and the
respective actuators in a single representative pump
or valve. This operation has been made to simplify the
complexity of the model to have a more manageable
but at the same time an essential system, in which the
control strategy of this study was applied. AGBAR
provided the demands episode of the network.
3.2 Control Objectives
Optimal control in water network deals with the
problem of generating flow control strategy from the
sources to the consumer areas to satisfy the demand
of water while optimizing performance goals such as
network safety volumes and flow control stability.
Thus, the following operational objectives should be
fulfilled by the distributed controllers by order of
priority:
Safety Term: the satisfaction of water demands
should be satisfied at any time instant, this is
guaranteed through the equality constraints of the
water mass balances at demand sectors. However,
some infeasibility avoidance mechanisms should be
introduced in the management of the tank volumes
such that this volume does not fall below a security
amount resulting in demands which cannot be
satisfied, this leads to the management of the tank
volumes above a specific security volume, which
ensures that the network can always supply the
demand flows.
Smooth Control Actions: pumps and valves
should operate smoothly to avoid large transients in
the pressurized pipes that can lead to their damage.
To obtain such smoothing effect, the MPC controller
includes in the objective function a term that
penalizes control signal variation
u(k).
Functional Requirements: the functional
requirements of this system are presented in Table I,
the control objectives are reflected in FR3, FR4, and
FR8. That means that the priority of the control is to
maintain the system inside the security levels, a
desirable reference is also considered but the priority
is FR3 and FR4. The latter one refers to a smooth
control, that means that control actions should
increase /decrease in small quantities.
3.3 Restriction and Considerations
The safety objective leads to the management of the
tank volumes above a specific security volume, which
ensures that the network can always supply the
demand flows. That is the minimum volume
restriction in tanks. A maximum safety level (to avoid
spills) should also be applied. Physical limits of
valves and pumps should be considered.
3.4 Definition of the Partitioning
For this case of study, the Barcelona DWN aggregate
network presented in Fig. 1, has been used. From this
figure, is clear that the network is comprised of 17
tanks (state variables), 61 actuators (26 pumping
stations and 35 valves), 11 nodes and 25 main sectors
of water demand (model disturbances). Nodes (of the
water network) correspond to the points where water
flows are merged or divided within the network.
Thus, the nodes represent mass balance relations and
are modelled as equality constraints related to inflows
and outflows of the nodes.
ICAART 2019 - 11th International Conference on Agents and Artificial Intelligence
562