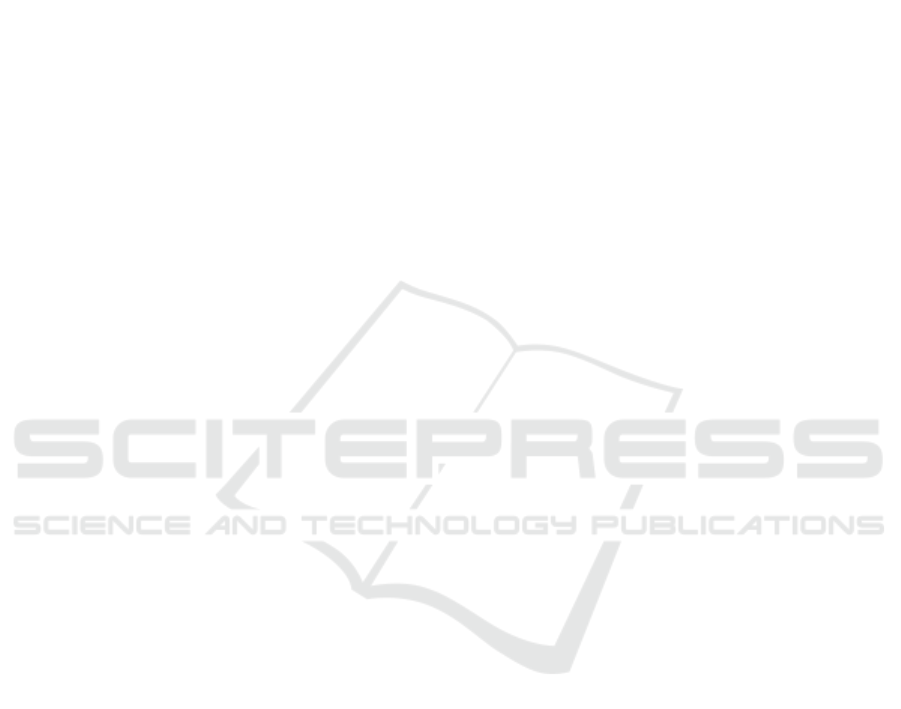
Microscale Optical Capture System for Digital Fabric Recreation
Raúl Alcain
1
, Carlos Heras
1
, Iñigo Salinas
1
, Jorge López
2
and Carlos Aliaga
2
1
Departamento de Ingeniería Eléctrónica y Comunicaciones, EINA, Universidad de Zaragoza,
C/María de Luna 1, 50018 Zaragoza, Spain
2
Desilico Labs, C/Tellez 24, 28007 Madrid, Spain
Keywords: Optical Capture, Fabric Recreation, Cloth Rendering, Divergence Beam.
Abstract: Synthetic images are ubiquitous in the world, being extensively used in advertising, industrial design and
prototyping. However, automatic digital reproduction of fabrics is still an open problem in industrial contexts,
due to the inherent complexity of cloth appearance. We present a system to capture images of fabrics at micron
resolution, lit from a set of collimated beams of LED luminaires distributed along the hemisphere. The system
ensures plane wavefront with a 1:1 magnification while minimizing self-occlusions. It also allows for specular
and diffuse light components separation through polarization, has a very accurate focusing system and a
shallow depth of field for depth extraction. We demonstrate the system is suitable for later extraction of
geometric and optical properties of the cloth at the fiber level, which is the main requisite for high fidelity
photo-realistic cloth rendering.
1 INTRODUCTION
Cloth rendering is a very active research area in
computer graphics, and is becoming of increasing
interest of many other fields. This is because digitally
reproducing the appearance of fabrics has many
applications not only in the entertainment industry but
also in the context of textile design and
manufacturing. However, the appearance of cloth is
the result of complex light scattering interactions that
occur within the micro-structures present at the fiber
level in textiles (Aliaga el al. 2017). Thus, micro scale
optical capturing systems are needed for accurately
extracting the geometric and optical properties of the
fabric that allow to reproduce the appearance under
any lighting condition, allowing rendering solutions
that reach the scale of fibers, (hundreds of
micrometers), to gain accuracy and predictive power
(Zhao et al. 2011).
Most existing devices are targeted to capture
generic surface-like (without volumetric structure)
materials at a millimeter scale, usually focused in
extracting the surface normals and its reflectance, the
latest in the form of a Bidirectional Reflectance
Distribution Function (BRDF) that can be spatially
varying (SV-BRDF). For this, digital cameras are
used to capture images with basically two
approaches. One of them uses multiple viewing and
lighting directions covering the hemisphere centered
in the normal of the material surface in order to
recover the Bidirectional Reflectance Distribution
Functions (BTF). The other one relies on a single
fixed view and multiple light sources. All these
systems commonly work with small magnification
relations (Schwartz et al. 2014), since they are
interested in recovering the SV-BRDF or the BTF
because they need a number of different viewing
directions. This implies many issues related to depth
of field at grazing angles, particularly critical when
trying to reach very fine resolutions. Thus, these kind
of solutions are very useful for mid-distance (a meter)
material appearance modeling, but do not provide
small enough pixel size to take the real details, and
therefore are not well suited when the goal is to
extract geometric and optical properties for later use
in a realistic rendering context, for instance in the
case of realistic fabrics.
Very few works present a microscale optical
capturing system. A recent example is the work of
Nam and colleagues (Nam et al. 2016). They
implement cameras with macro lens, leading to very
short optical working distances that allow reducing
the dimensions of the system up to a few tens of
millimeters. Their system uses multiple LEDs and a
fixed viewing direction, working with up to 5:1
magnification. All components are built in a 40 mm
114
Alcain, R., Heras, C., Salinas, I., López, J. and Aliaga, C.
Microscale Optical Capture System for Digital Fabric Recreation.
DOI: 10.5220/0007356201140119
In Proceedings of the 7th International Conference on Photonics, Optics and Laser Technology (PHOTOPTICS 2019), pages 114-119
ISBN: 978-989-758-364-3
Copyright
c
2019 by SCITEPRESS – Science and Technology Publications, Lda. All rights reserved