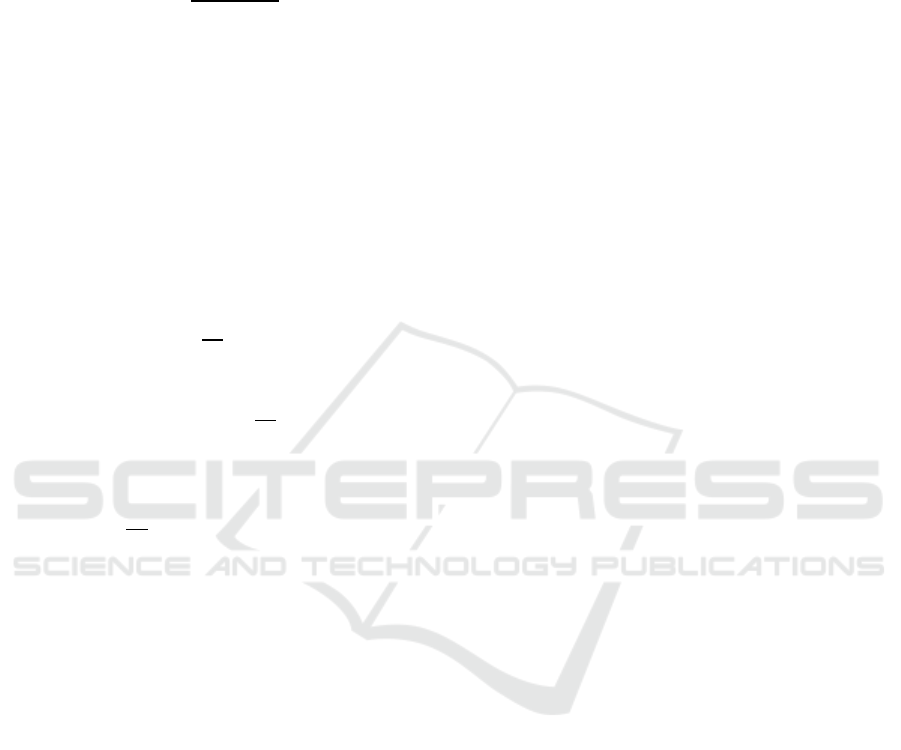
where c is natural number. We evenly discretize the
test function at 30 points to reconstruct by using the
eigenvectors. Figure 4 illustrates the mean square
error versus the number of eigenvectors. The
normalized mean square error is defined by
where
is the sum in Eq. (26) up to , is the
original vector and
is the
-norm. From Fig. 4,
we can see that the error decreases with increasing
number of eigenvectors used in the expansion.
Next, let us consider another following test function.
where indicates noise and is a normally distributed
deviate with zero mean and unit variance. To measure
the effect of noise on the function, we use the signal-
to-noise ratio (SNR) (Trussel, 2008). This is usually
defined as the ratio of signal power
, to noise
power
,
and in decibels
In
, the function power is usually estimated by the
simple summation
where
is the mean of the function. Figure 5
illustrates the mean square error versus the number of
eigenvectors with noise. The SNR in example 1
(Ex.1) is 18.019354, example 2 (Ex. 2) is 8.476929
and example 3 (Ex. 3) is 4.039954. From Fig. 5, we
can see that the original test function is reconstructed
in the state that is almost perfection if SNR increases.
In general, it is difficult for the small value of SNR to
reconstruct original test function completely. Figure
6 illustrates the mean square error versus the number
of eigenvectors for the phase without noise. The mean
square error is defined by
From Fig. 6, we can see also that the error decreases
with increasing number of eigenvectors used in the
expansion for the phase.
5 CONCLUSIONS
Band-limited effects with respect to Fourier
transform have already been investigated and well
known. However, those with respect to Fresnel
transform have not been studied and revealed
sufficiently. We have investigated the band-limited
effect in Fresnel transform plane. For that, we have
sought the function that its total power in finite
Fresnel transform plane is maximized, on condition
that an input signal is zero outside the bounded
region. We have shown that this leads to the
eigenvalue problems of Fredholm integral equation of
the first kind. It is important to reveal the
mathematical properties of the integral equation for
finite Fresnel transform. Orthogonal eigenfunctions
are derived from its properties. Orthogonal functional
systems are significant tools in analysing a diffraction
image. We have also shown that the eigenfunctions
corresponding to distinct eigenvalues have dual
orthogonal property. These functional systems and its
properties show clearly the relation between
functions and their Fresnel transforms. It is difficult
in general to seek the strict solution of the integral
equation. So we desired to seek the approximate
solution in practical exact accuracy. Furthermore, we
applied it to the problem of approximating a function
and evaluated the error. We confirmed the validity of
the eigenvectors for finite Fresnel transform by
computer simulations.
In this study, there are many parameters,
especially, the band-limited areas , the wave
number and the normal distance . It is necessary to
consist of orthogonal functional systems with the
optimal parameters for finite Fresnel transform in
application of an optical system. Moreover, in
general, the matrix given by discretizing the kernel of
the integral equation is not the Hermitian matrix. If
so, it is difficult to compute accurately all eigenvalues
and eigenvectors. It is also necessary to consider other
computational methods for this. Although the kernel
function was discretizing at 30 point, it is necessary
to increase the number of sampling points. Although
we considered only one dimensional Fresnel
transform, it is necessary to derive the integral
equation for the two dimensional Fresnel transform.
These become the future problems. Theoretically, it
is important to search for a spectral representation of
finite Fresnel transform which are defined as a
bounded linear operator in Hilbert space.
REFERENCES
Aoyagi, N., 1973. Theoretical study of optical Fresnel
transformations. Dr. Thesis, Tokyo Institute of
Technology, Tokyo
Aoyagi, N., Yamaguchi, S., 1973a. Functional analytic
formulation of Fresnel diffraction. Jpn. J. Appl. Phys.
12, 336-370
Aoyagi, N., Yamaguchi, S., 1973b. Generalized Fresnel
transformations and their properties. Jpn. J. Appl. Phys.
12, 1343-1350
PHOTOPTICS 2019 - 7th International Conference on Photonics, Optics and Laser Technology
152