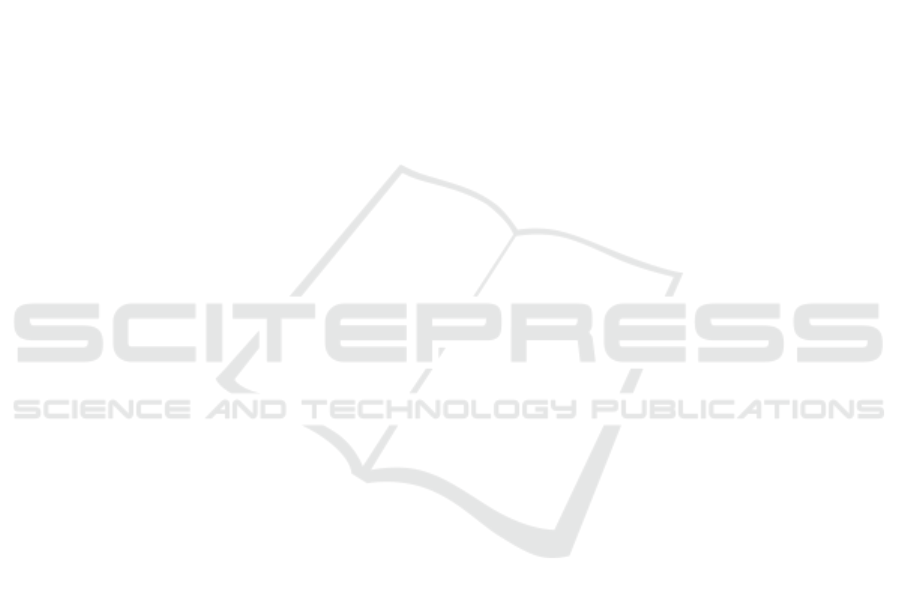
Long-Range (>100km) Distributed Vibration Sensor based on
Φ-OTDR Technique with Spread Amplification and Detection of
Probe Pulses
David Sanahuja
1
, Javier Preciado
2,3
, Jesús Subías
1
, Carlos Heras
2
, Lucía Hidalgo
2
, Iñigo Salinas
2
,
Pascual Sevillano
3
, Juan José Martínez
3
and Asier Villafranca
3
1
Departamento de Física Aplicada, Ciencias, Universidad de Zaragoza, Pedro Cerbuna 12, 50009 Zaragoza, Spain
2
Departamento de Ingeniería Electrónica y Comunicaciones, EINA, Universidad de Zaragoza, María de Luna 1,
50018 Zaragoza, Spain
3
Aragón Photonics Labs (APL), Prado 5, 50009 Zaragoza, Spain
a.villafranca}@aragonphotonics.com
Keywords: Optical Fiber, Fiber-Optic Sensor, Distributed Sensor, Rayleigh Scattering, Coherent Optical Time Domain
Reflectometry (Φ-OTDR), Long-Range Distributed Sensing, Modulation Instability, Fading.
Abstract: This paper presents a set of results to demonstrate a long-range (>100km) distributed vibration sensor (DAS)
based on the Coherent Optical Time Domain Reflectometry (Φ-OTDR) technique using distributed
amplification of the probe pulses and detection of the backscattered traces, which demonstrates great
capability to achieve long-range distances with great sensitivity. In this case, optical amplifiers have been
placed along the sensing optical fiber, each one followed by a detection stage. Results for some traces detected
in each of the spans along the sensing fiber, and some measurements of stimuli produced by a vibration at the
end of each of the sections of the sensing fiber, are showed here. This work, framed in the project SACOH
(Long-range Distributed Vibration Sensing by Coherent Rayleigh Backscattering), has been carried out in
collaboration between the University of Zaragoza and the company APL (Aragón Photonics Labs).
1 INTRODUCTION
Distributed vibration sensing technologies have
greatly expanded their use in the recent years due to
the wide range of applications that they offer (Bao
and Chen, 2012). Among these applications
(monitoring of the integrity of civil engineering
structures and power plants, detection of leaks,
control of railways, traffic control...), it stands out
perimeter surveillance of infrastructures with a large
perimeter to be monitored. Surveillance strategies
based on conventional technologies (video
surveillance or conventional motion sensors…) are
no longer viable after a few kilometers due to the
large increase in their cost, because of the enormous
growth in complexity and in management problems
of monitoring such infrastructures when the length to
be monitored is increased. Therefore, the
development of new long-range detection strategies
such as those based on distributed optical sensing
technologies, in particular distributed vibration
sensing techniques, has great interest.
Distributed optical sensing technologies use
different measurement strategies, and their operation
is based on the use of a wide variety of physical
phenomenologies, mainly Rayleigh, Brillouin and
Raman optical dispersion. Systems based on Raman
and Brillouin scattering are mostly employed in the
monitoring of the integrity of large structures (Barrias
et al., 2016) through temperature and mechanical
stress measurements, while systems based on
Rayleigh scattering are mostly used in dynamic
scenarios typical of perimeter surveillance (Rao et al.,
2008).
Measurement systems based on coherent optical
reflectometry base their operation on Rayleigh
backscattering to detect disturbances due to stimuli
(vibrations or pressure changes) produced on the fiber
environment, by sensing local phase changes
(Muanenda, 2018). A pulsed laser with a highly stable
emission frequency and high coherence is used to
196
Sanahuja, D., Preciado, J., Subías, J., Heras, C., Hidalgo, L., Salinas, I., Sevillano, P., Martínez, J. and Villafranca, A.
Long-Range (>100km) Distributed Vibration Sensor based on F-OTDR Technique with Spread Amplification and Detection of Probe Pulses.
DOI: 10.5220/0007386401960200
In Proceedings of the 7th International Conference on Photonics, Optics and Laser Technology (PHOTOPTICS 2019), pages 196-200
ISBN: 978-989-758-364-3
Copyright
c
2019 by SCITEPRESS – Science and Technology Publications, Lda. All rights reserved