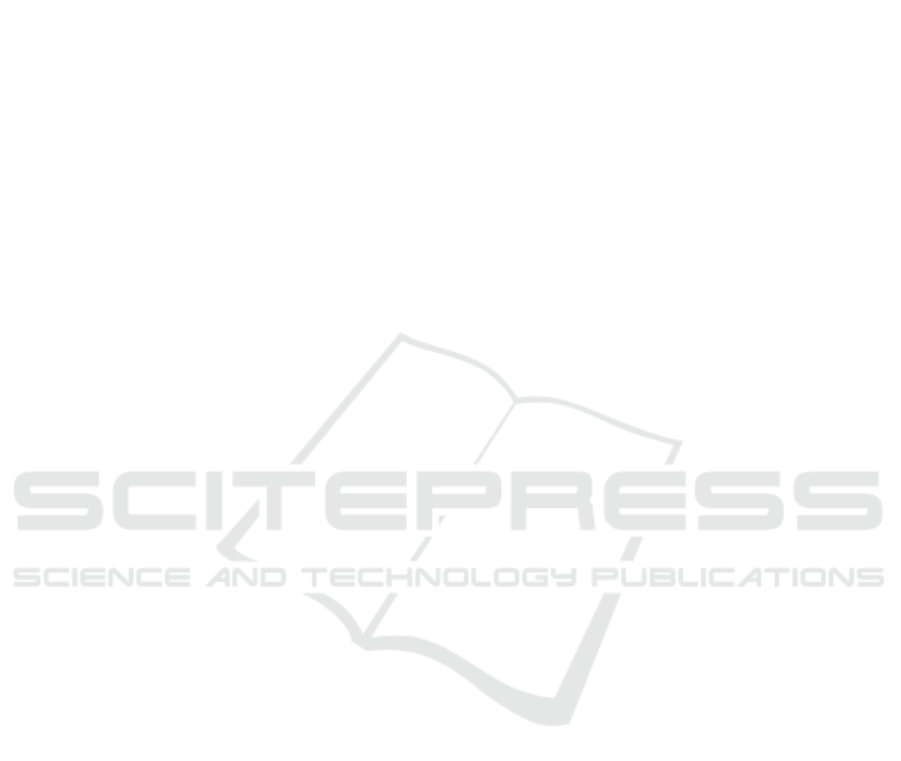
Solar Pumped Lasers for Free Space Laser Communication
Changming Zhao, Haiyang Zhang, Zhe Guan, Zitao Cai, Dongbing He and Yongheng Wang
Beijing Institute of Technology, Beijing, China
Keywords: Solar Pumped Lasers, Multi-Frequency, Free Space Laser Communication.
Abstract: Solar Pumped Lasers (SPL) is a kind of lasers that can transform solar light into laser directly, with the
advantages of least energy transform procedure, higher energy transform efficiency, higher reliability, and
longer lifetime, which is suitable for use in unattended space system, for solar light is the only form of energy
source in space. In order to exploring the possibility of using SPL for free space laser communication, multi-
frequency SPL is investigated and solar pumped laser amplification is initiated. The first demonstration of
SPL used in free space laser communication is also conducted in our group.
1 INTRODUCTION
Solar pumped laser (SPL) is a special kind of lasers,
that can transform broad-band, incoherent solar light
into narrow-band, coherent laser directly, with the
advantages of least energy transform procedure,
higher energy transform efficiency, higher reliability
and longer lifetime. It is suitable for application in
unattended space system, such as space laser
communication, space to earth wireless power
transmission and space laser propulsion(Mori et
al.,2006; Guan et al.,2017; Oliveira et al.2016; Yabe
et al., 2008).
SPL has a comparable long research history as
lasers itself. Shortly after lasers was invented in 1960,
solar light was considered as the pumping source of
solid state lasers, for all solid state lasers were light-
pumped and solar light is the mostly common light
source we encountered in daily life. SPL was first
demonstrated by Z. J. Kiss from RCA Laboratories in
1963(Kiss et al., 1963), a Dy
2+
: CaF
2
crystal was
cooled in liquid nitrogen and pumped by solar light.
Shortly thereafter, systems using solar light to pump
different kinds of laser mediums were considered.
Among various laser mediums (solid, liquid and
gaseous), solid state lasers appear to be most
competitive because of stable performance, lower
pumping threshold, and potential efficient of solar-to-
laser power conversion. The first Solar-pumped solid
laser was reported by Young from the American
Optical Company in 1966(Young, 1966), 1W of
continuous wave laser output was obtained at room
temperature via a Nd:YAG crystal. In 1988, Weksler
and Shwartz from Weizmann Institute of Science,
Israel, through a compound parabolic concentrator
(CPC) obtained 60 W CW output power of laser from
a Nd:YAG rod with a slope efficiency about
2%(Weksler and Shwartz, 1998) . In 2007, T. Yabe
from Tokyo Institute of Technology demonstrated an
18.7 W/m
2
laser output from a Cr
3+
, Nd
3+
co-doped
YAG ceramic with Fresnel lens as the primary solar
light concentrator, corresponding to a total slope
efficiency of 2.9%(Yabe et al., 2007). In 2011, Liang
from Universidade NOVA de Lisboa, Portugal, 19.3
W/m
2
laser collection efficiency was achieved from a
Φ425mm Nd:YAG rod, which is pumped by a 0.64
m
2
Fresnel lens(Liang and Almeida, 2011). T. Yabe’s
group reported a SPL with 120 watts CW output in
2012(Dinh et al., 2012). They used a 4 m
2
Fresnel lens
as first solar energy collector and a Φ6mm Nd:YAG
rod, the collection efficiency was 30W/m
2
. For
further thermal management, grooved Nd:YAG laser
rod was firstly used in SPL in 2014(Xu et al., 2014).
Zhao´s group from Beijing Institute of Technology
achieved 27 W laser power by utilizing a Φ695mm
Nd:YAG grooved rod pumped by a 1.03 m
2
Fresnel
lens, corresponding to a slope efficiency of 9.0%. The
grooved Nd:YAG rod offered better heat dissipation
and reduced the thermal lens effect, compared with
that of unpolished rod, leading to a superior efficiency
and beam quality. In 2017, D. Liang and J. Almeida
used the heliostat-parabolic mirror system to pump a
Nd:YAG rod and obtained a collection efficiency of
31.5 W/m
2
(Liang et al., 2017). The highest collection
efficiency of 32.1W/m
2
, for the time being, was
achieved by Beijing Institute of Technology, using a
268
Zhao, C., Zhang, H., Guan, Z., Cai, Z., He, D. and Wang, Y.
Solar Pumped Lasers for Free Space Laser Communication.
DOI: 10.5220/0007569702680275
In Proceedings of the 7th International Conference on Photonics, Optics and Laser Technology (PHOTOPTICS 2019), pages 268-275
ISBN: 978-989-758-364-3
Copyright
c
2019 by SCITEPRESS – Science and Technology Publications, Lda. All rights reserved