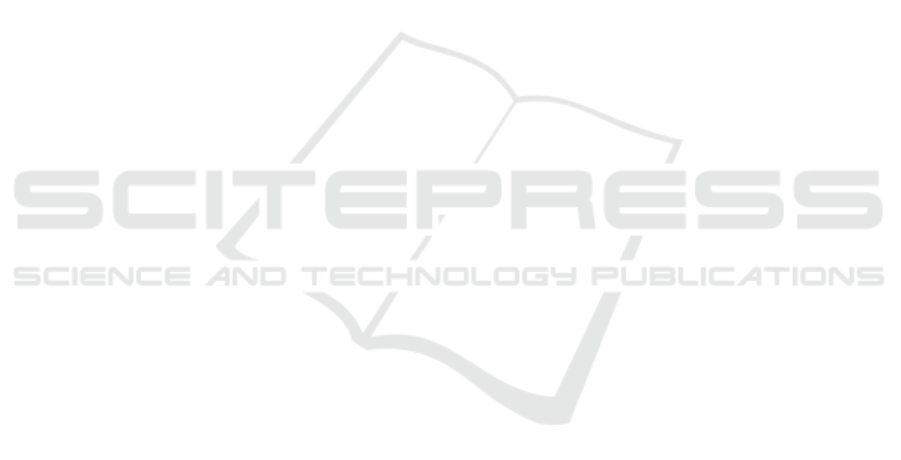
edpr-celebrates-ninth-edition-university-challenge-
awards-spain .
Altan, B. D. and Atılgan, M. (2012). A study on increas-
ing the performance of savonius wind rotors. Journal
of Mechanical Science and Technology, 26(5):1493–
1499.
Arteaga-L
´
opez, E.,
´
Angeles-Camacho, C., and Ba
˜
nuelos-
Ruedas, F. (2019). Advanced methodology for feasi-
bility studies on building-mounted wind turbines in-
stallation in urban environment: Applying cfd analy-
sis. Energy, 167:181–188.
Berrisford, P., Dee, D., Fielding, K., Fuentes, M., Kallberg,
P., Kobayashi, S., and Uppala, S. (2009). The era-
interim archive. ERA report series, (1):1–16.
Bhatti, T., Kothari, D., et al. (2003). A new vertical axis
wind rotor using convergent nozzles. In Power Engi-
neering, 2003 Large Engineering Systems Conference
on, pages 177–181. IEEE.
Bogle, I. (2011). Integrating wind turbines in tall buildings.
CTBUH Journal, 4:30–33.
Chastas, P., Theodosiou, T., Bikas, D., and Kontoleon, K.
(2017). Embodied energy and nearly zero energy
buildings: A review in residential buildings. Proce-
dia environmental sciences, 38:554–561.
Chong, W., Naghavi, M., Poh, S., Mahlia, T., and Pan, K.
(2011). Techno-economic analysis of a wind–solar
hybrid renewable energy system with rainwater col-
lection feature for urban high-rise application. Applied
Energy, 88(11):4067–4077.
Chong, W., Pan, K., Poh, S., Fazlizan, A., Oon, C.,
Badarudin, A., and Nik-Ghazali, N. (2013). Perfor-
mance investigation of a power augmented vertical
axis wind turbine for urban high-rise application. Re-
newable Energy, 51:388–397.
Dilimulati, A., Stathopoulos, T., and Paraschivoiu, M.
(2018). Wind turbine designs for urban applications:
A case study of shrouded diffuser casing for turbines.
Journal of Wind Engineering and Industrial Aerody-
namics, 175:179–192.
Kim, S. and Cheong, C. (2015). Development of low-noise
drag-type vertical wind turbines. Renewable energy,
79:199–208.
Manwell, J. F., McGowan, J. G., and Rogers, A. L. (2010).
Wind energy explained: theory, design and applica-
tion. John Wiley & Sons.
Mertens, S. (2006). Wind energy in the built environment:
concentrator effects of buildings.
Mohamed, M., Janiga, G., Pap, E., and Th
´
evenin, D. (2010).
Optimization of savonius turbines using an obstacle
shielding the returning blade. Renewable Energy,
35(11):2618–2626.
Oerlemans, S., Sijtsma, P., and L
´
opez, B. M. (2007). Loca-
tion and quantification of noise sources on a wind tur-
bine. Journal of sound and vibration, 299(4-5):869–
883.
Olauson, J. (2018). Era5: The new champion of wind power
modelling? Renewable energy, 126:322–331.
Park, J., Jung, H.-J., Lee, S.-W., and Park, J. (2015). A new
building-integrated wind turbine system utilizing the
building. Energies, 8(10):11846–11870.
Penalba, M., Ulazia, A., Ibarra-Berastegui, G., Ringwood,
J., and S
´
aenz, J. (2018). Wave energy resource varia-
tion off the west coast of ireland and its impact on re-
alistic wave energy converters’ power absorption. Ap-
plied Energy, 224:205–219.
Rabanal, A., Ulazia, A., Ibarra-Berastegi, G., S
´
aenz, J.,
Elosegui, U., et al. (2018). Midas: A benchmarking
multi-criteria method for the identification of defec-
tive anemometers in wind farms. Energies, 12(1):1–
19.
Roy, S. and Saha, U. K. (2013). Review on the numeri-
cal investigations into the design and development of
savonius wind rotors. Renewable and Sustainable En-
ergy Reviews, 24:73–83.
SeaHawk (2017). SeaHawk-Desert Power Savonious tur-
bine. Desert Power.
Smith, R. F. and Killa, S. (2007). Bahrain world trade cen-
ter (bwtc): the first large-scale integration of wind tur-
bines in a building. The structural design of tall and
special buildings, 16(4):429–439.
Tong, C. W., Zainon, M., Chew, P. S., Kui, S. C., Keong,
W. S., and Chen, P. K. (2010). Innovative power-
augmentation-guide-vane design of wind-solar hybrid
renewable energy harvester for urban high rise appli-
cation. In AIP Conference Proceedings, volume 1225,
pages 507–521. AIP.
Ulazia, A. (2016). Multiple roles for analogies in the gen-
esis of fluid mechanics: How analogies can cooper-
ate with other heuristic strategies. Foundations of Sci-
ence, 21(4):543–565.
Ulazia, A., Penalba, M., Ibarra-Berastegui, G., Ringwood,
J., and Sa
´
enz, J. (2017a). Wave energy trends over the
bay of biscay and the consequences for wave energy
converters. Energy, 141:624–634.
Ulazia, A., Penalba, M., Rabanal, A., Ibarra-Berastegi, G.,
Ringwood, J., and S
´
aenz, J. (2018). Historical evolu-
tion of the wave resource and energy production off
the chilean coast over the 20th century. Energies,
11(9):2289.
Ulazia, A., Saenz, J., and Ibarra-Berastegui, G. (2016).
Sensitivity to the use of 3dvar data assimilation in a
mesoscale model for estimating offshore wind energy
potential. a case study of the iberian northern coast-
line. Applied Energy, 180:617–627.
Ulazia, A., S
´
aenz, J., Ibarra-Berastegui, G., Gonz
´
alez-Roj
´
ı,
S. J., and Carreno-Madinabeitia, S. (2017b). Using
3dvar data assimilation to measure offshore wind en-
ergy potential at different turbine heights in the west
mediterranean. Applied energy, 208:1232–1245.
Venables, W. N., Smith, D. M., and Team, R. C. (2018). An
introduction to r-notes on r: A programming environ-
ment for data analysis and graphics.
Wong, K. H., Chong, W. T., Sukiman, N. L., Poh, S. C.,
Shiah, Y.-C., and Wang, C.-T. (2017). Performance
enhancements on vertical axis wind turbines using
flow augmentation systems: A review. Renewable and
Sustainable Energy Reviews, 73:904–921.
WWEA, A. (2016). WWEA released latest Global Small
Wind Statistics. WWEA.
Zemamou, M., Aggour, M., and Toumi, A. (2017). Review
of savonius wind turbine design and performance. En-
ergy Procedia, 141:383–388.
SMARTGREENS 2019 - 8th International Conference on Smart Cities and Green ICT Systems
178