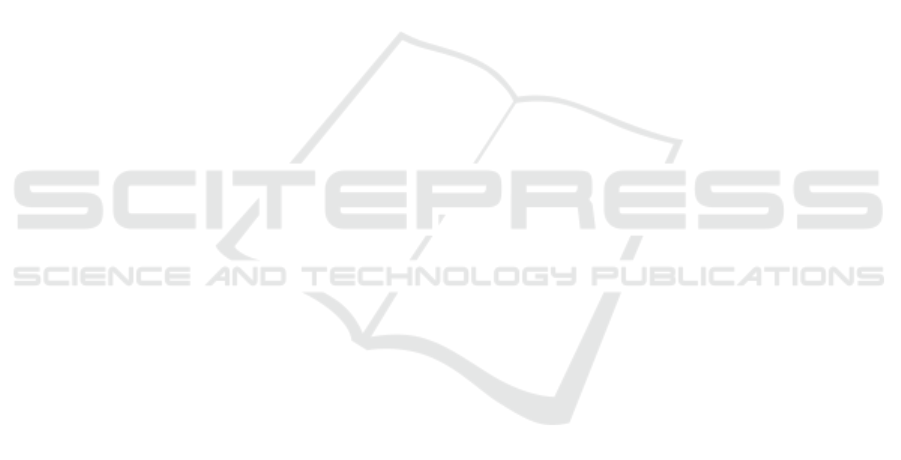
plants, which went in operation in 2015 and 2016, re-
main base load. This only changes when prices are
increased to 75 €/tCO
2
(figure 3d).Hence, stating one
CO
2
price that is needed for a shift from coal to gas is
too simplistic; it is depending on the entire generating
mix, and the relative age of the different facilities. This
varies from country, providing a strong argument for
national policies for CO
2
taxes on top of the European
level policies. Furthermore, this shows that even high
CO
2
prices are not able to push all coal-fired power
plants out of the merit order, despite their higher
emissions. Either very high CO
2
prices (from 100
euro per tonne), or additional measures are needed if
policy makers decide these emissions should be de-
creased.
4 CONCLUDING REMARKS
In this paper, we presented a method for designing
marginal emission profiles for a specific country
based on its generation merit order and applied this
for the case study of the Netherlands. The value of
this approach can be understood from two perspec-
tives. From a bottom-up perspective, consumers may
reconsider the scheduling of their electricity demand.
The operation of shiftable loads, such as electric ve-
hicles, wet appliances and stationary storage devices
can be scheduled considering the minimization of
CO
2
emissions, in addition to cost, if the demand can
be shifted to periods with cleaner periods. This can be
from coal to gas in the nearby future, but also in a
more distant future from periods with fossil-fuel
based power plants operating at the margin to periods
where renewables are operating at the margin. From
a top-down perspective, the approach might help to
better determine the impact of implementing renewa-
bles in the generation mix, and for determining ade-
quate CO
2
prices to enforce a shift from coal to gas.
ACKNOWLEDGEMENTS
This project is part of the PVProsumers4Grid Project,
which received funding from the European Union’s
Horizon 2020 research and innovation programme
under grant agreement No 764786. Furthermore, this
work has received funding in the framework of the
joint programming initiative ERA-Net Smart Grids
Plus as part of the CESEPS project, as well as from
TKI Urban Energy (Project: B-DER, contract number
1621404).
REFERENCES
Afman, M. R., and Wielders, L. M. L. (2014).
Achtergrondgegevens stroometikettering 2013. Delft.
Arcadis. (2009). Passende beoordeling Diemen 34 Natura
2000-gebied Markermeer & IJmeer. Retrieved from
http://www.commissiemer.nl/docs/mer/p20/p2097/209
7-026vergunningaanvraag003.pdf
Bettle, R., Pout, C. H., and Hitchin, E. R. (2006).
Interactions between electricity-saving measures and
carbon emissions from power generation in England
and Wales. Energy Policy, 34(18), 3434–3446.
https://doi.org/10.1016/j.enpol.2005.07.014
Biggar, D. R., and Hesamzadeh, M. R. (2014). The
Economics of Electricity Markets. The Economics of
Electricity Markets (Vol. 9781118775). https://doi.org/
10.1002/9781118775745
Brouwer, A. S., van den Broek, M., Seebregts, A., and
Faaij, A. (2015). Operational flexibility and economics
of power plants in future low-carbon power systems.
Applied Energy, 156, 107–128. https://doi.org/
10.1016/j.apenergy.2015.06.065
CBS. (2017). Ketelkolen ; invoerprijs uit niet EU-landen.
Retrieved April 19, 2018, from http://statline.cbs.nl/
StatWeb/publication/?VW=T&DM=SLNL&PA=3721
5&D1=2&D2=a&HD=120417-1100&HDR=T&STB=
G1
CBS. (2018a). Hernieuwbare elektriciteit; productie en
vermogen. Retrieved from http://statline.cbs.nl/
Statweb/publication/?DM=SLNL&PA=82610ned&D1
=0%2C7&D2=a&D3=a&HDR=T&STB=G1%2CG2&
VW=T
CBS. (2018b). Rendementen en CO2-emissie elektriciteits-
productie 2016. https://doi.org/10.1023/A:102023833
0817
Croezen. (2016). Analyse conclusies en uitgangspunten
berekening Stadsverarming. Delft.
D66. (2015). Kolendeal Energieakkoord : Emissiereductie
en verduurzaming kan en moet. Retrieved August 8,
2018, from https://d66.nl/content/uploads/sites/2/2015/
03/DELTA-Kolendeal-Energieakkoord.pdf
Enipedia. (2018). Den Haag Powerplant. Retrieved August
9, 2018, from http://enipedia.tudelft.nl/wiki/
Den_Haag_Powerplant
ENTSO-E. (2019a). Day-ahead Prices. Retrieved March 8,
2019, from https://transparency.entsoe.eu/transmis
sion-domain/r2/dayAheadPrices/show
ENTSO-E. (2019b). Installed Capacity Per Production
Unit. Retrieved March 30, 2018, from
https://transparency.entsoe.eu/generation/r2/installedC
apacityPerProductionUnit/show
Graus, W., and Worrell, E. (2011). Methods for calculating
CO2 intensity of power generation and consumption: A
global perspective. Energy Policy, 39(2), 613–627.
https://doi.org/10.1016/j.enpol.2010.10.034
Harmsen, R., and Graus, W. (2013). How much
CO2emissions do we reduce by saving electricity? A
focus on methods. Energy Policy, 60, 803–812.
https://doi.org/10.1016/j.enpol.2013.05.059
SMARTGREENS 2019 - 8th International Conference on Smart Cities and Green ICT Systems
192