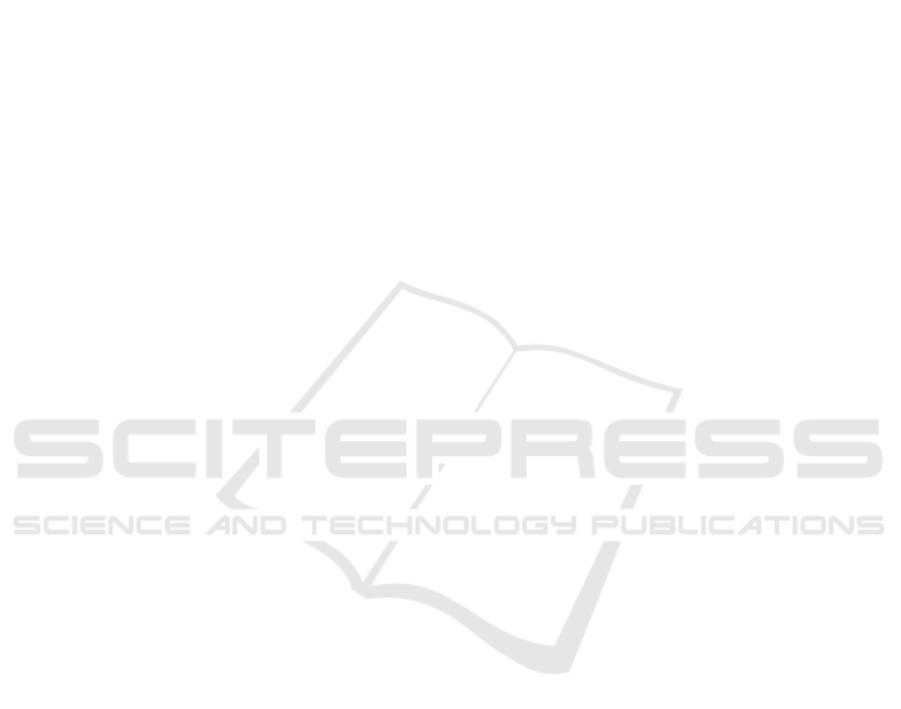
Simulation Effects on the Optical Response of Gold Nanoparticles
Tanaporn Leelawattananon
1
and Suphamit Chittayasothorn
2
1
Department of Physics, Faculty of Science, King Mongkut’s Institute of Technology Ladkrabang, Bangkok, Thailand
2
Department of Computer Engineering, Faculty of Engineering, King Mongkut’s Institute of Technology Ladkrabang,
Bangkok, Thailand
Keywords: Localized Surface Plasmon Resonances, Gold Nanoparticles, Optical Properties, Finite Element Method.
Abstract: In this research work, we use simulation models for the investigation of the sizes and shapes of gold
nanoparticles on BK-7 substrate base, which affect the optical characteristics in a large spectral range of the
gold nanoparticles. Linearly polarized light with wavelengths of 300 – 800 nm are specified as the impact
light on gold nanostructures. We try to find the suitable wavelength of the impact light when localized
surface plasmon resonance takes place. The gold nanoparticles are spherical, elliptical oval, and 10nm x 40
nm block-shape and have their aspect ratio similar to the nanorod shape nanoparticles used in other
experiments. The results are useful for the development of plasmonic complex nanostructure with tunable
surface plasmon resonances which generate heat and have potential applications in medical thermal
therapy.
1 INTRODUCTION
According to technological advancement, metals
which have structures at the nanometer level can be
manufactured. This advancement attracts attention
of physicists, chemists, material scientists, and
biologists which are interested in the surface
plasmon phenomenon. Surface Plasmon Resonance
(SPR) is the phenomenon which takes place when
there are excitations of free electrons at the interface
between an electrical conductor and a dielectric
layer thus create evanescent electromagnetic waves.
The excitation of Surface Plasmon waves leads to
the occurrence of other phenomena, including the
optical responses of materials. Plasmonics in the
material structures at the nanometer level find
applications in the surface-enhanced sensing and the
measuring of intra molecular distances in molecules.
The optical response of metallic nanoparticles
(NPs) can be adjusted by the controlling of the size
and shape of the particles and also the environment.
This knowledge initiates research areas such as
Surface Plasmon based Photonics or Plasmonics (G.
Alexandre Brolo, 2012). There have also been new
techniques in the synthesis of nano particles which
aim at the creation of nanoparticles, which have
adequate size and shape for excitation of the Surface
Plasmon waves (Z. Ying et.al., 2018). These surface
plasmon waves can be employed in magneto-optic
applications. They also help improve the efficiency
of surface-enhanced sensing and spectroscopy,
which find applications in bio detection and
chemical therapy (Z. Zhang et.al., 2014).
It has been recently found that the shape of the
nanoparticles affects the optical response of the
particles. Researches have been conducted to study
optical characteristics of nanoparticles and to find
out the effects of sizes and shapes of these
nanoparticles. Shapes include spherical, cube, and
other polyhedrons (Y. Wang et. Al., 2013) ( M.
Chen et.al., 2017). The more nanoparticle becomes
sphere-like, the more the main surface plasmon
resonance is red-shifted where the dependence of the
position of the resonances is analytically explained
in terms of their aspect ratio (Cecilia Noguez,
2007). The development of plasmonic complex
nanostructure with tunable surface plasmon
resonances results in a higher heat generation which
finds applications in medical thermal therapy (H.
Vahid et.al., 2018) (K. Jiang et.al., 2013).
In this project, we simulate the Surface Plasmon
Resonance phenomenon of gold nanoparticles which
have different shapes and sizes and have been
employed to align 1D structures. The finite element
method (FEM) is used in the analysis and
simulations. The simulation results show plasmon
wave electric fields when using these gold
360
Leelawattananon, T. and Chittayasothorn, S.
Simulation Effects on the Optical Response of Gold Nanoparticles.
DOI: 10.5220/0007959603600367
In Proceedings of the 9th International Conference on Simulation and Modeling Methodologies, Technologies and Applications (SIMULTECH 2019), pages 360-367
ISBN: 978-989-758-381-0
Copyright
c
2019 by SCITEPRESS – Science and Technology Publications, Lda. All rights reserved