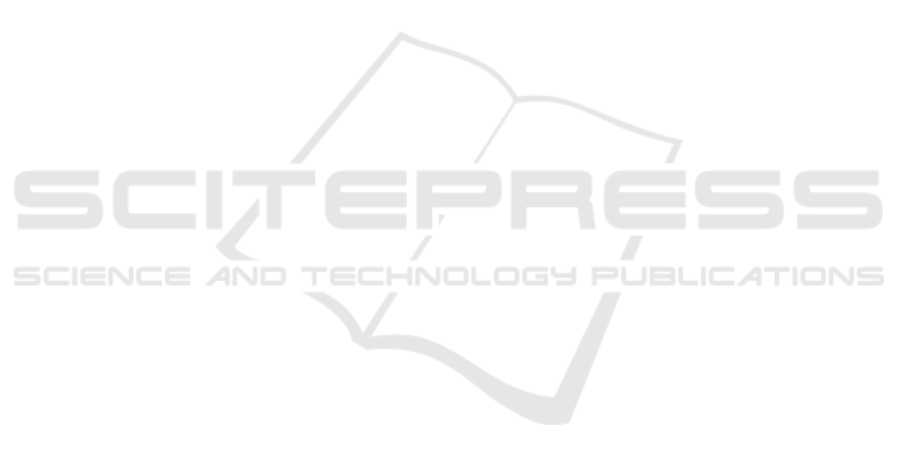
Ceccarelli, S., Erskine, W., Humblin, J., Brando, S. 2007.
Genotype by environment interaction and international
breeding program. http://www.icrisat.com.
Celik, O., Atak, C. 2011. Evaluation of the proline
accumulation and Δ'-pyrroline-5-carboxylate
synthetase (P5CS) gene expression during salinity
stress in two soybean (Glycine max L. Merr.) Varieties.
Plant Molecular Biology Reporter, 30: 566-577.
Ermawati, N. 2011. Ermawati, N. 2011. Isolation and
characterization of intrinsic membrane protein-coding
genes of plants halofit tonoplas Salicornia herbacea.
Journal of Basic Science, 12 (1): 23-29.
Gao, S. Q, Chen, M., Xu, Z. S., Zhao, C. P., Li, L., Xu, H.,
Tang, Y., Zhao, X., Ma, Y.Z. 2011. The soybean
GmbZIP1 transcription factor enhances multiple abiotic
stress tolerances in transgenic plants. Plant Molecular
Biology, 75: 537-553.
Ghassemi-Golezani, K., Taifeh-Noori, M. 2011. Soybean
performance under salinity stress. soybean. In
Biochemistry, Chemistry and Physiology. Intechopen,
631-642.
Hardiarto, T. 2010. Transcription factors of OsDREB1A
and OsDREB1B in rice. Research and Development
Center for Biotechnology and Genetic Resources
Agriculture. Institution of Agricultural Research and
Development, Ministry of Agriculture. http://biogen.
litbang.pertanian.go.id/index.php/2010/11/faktor-
transkripsi-osdreb1a-dan-osdreb1b-pada-padi/
Idris, U. M., Yakop, Farida, N. 2011. Progress in mass
selection of local corn cultivar (kebo) intercropping
with peanuts after one selection cycle. Kemajuan
seleksi massa pada jagung kultivar lokal kebo setelah
satu siklus seleksi dalam pertanaman tumpangsari
dengan kacang tanah. Crop Agronomy, 4 (2): 37-42.
Lan, C. H., Nguyen, T. A., Nguyen, V. T. T., Nguyen, H.
H., Mau, C. H. 2011. The characterization of the
GmDREB5 gene isolated from soybean cultivar
XanhTiendai, Vietnam. In Proceedings of International
Conference on Biology, Environment and Chemistry 1:
354-358.
Li, W. F., Wong, F. L., Tsai, S, N., Phang, T. H., Shao, G.,
Lam, H. M. 2006. Tonoplast-located GmCLC1 and
GmNHX1 from soybean Enhance NaCl tolerance in
transgenic bright yellow (BY) -2 cells.Plant, Cell and
Environment, 29: 1122-1137.
Liao, H., Wong, T., Phang, T. H., Cheung, M. Y., Li, W. F.,
Shao, G., Yan X., Lam, H. M. 2003. GmPAP3, a novel-
like purple acid phosphatase gene in soybean induced
by NaCl stress but not phosphorus deficiency. Gene,
318: 103- 111.
Marquez-Ortiz, J. J., Lamb, J. F. S., Johnson, L.D., Barnes,
D.K., and Stucker, R.E. 1999. Heritability of crown
properties in alfalfa. Crops Science, 39: 38 -43.
Munns, R. 2005. Genes and salt tolerance: bringing them
together. New Phytologist, 167 (3): 645-663.
Passiora, J. B., 1996. Drought and drought tolerance. Plant
Growth Regulation, 20: 79-83.
Pathan, M. S., Lee, J. D., Shannon, J. G., Nguyen, H. T.
2007. Recent advances in breeding for drought and salt
stress tolerance in soybean. In Advances in molecular
breeding toward drought and salt tolerant crops
Springer, Dordrecht. 739-773.
Peng, H., Feng, Y., Zhang, H., Wei, X., Liang, S. 2012.
Molecular cloning and characterization of genes coding
for Glycine- and Proline-Rich Proteins (GPRPs) in
Soybean. Plant Molecular Biology Reporter, 30: 566-
577.
Phang, T. H., Shao, G., Lam, H. M. 2008. Salt tolerance in
soybean. Journal of Integrative Plant Biology, 50(10),
1196-1212.
Roy, D., 2000. Plant breeding: Analysis and exploitation of
variation. Narosa Publishing House Calcutta.
Santoso, T. J., Abdullah, B., Carsono, N., Apriana, A.,
Sisharmini, A., Trijatmiko, K. R. 2012. Synergy and
stability expression of the Os F1 and OsDREB1A genes
in the transgenic Ciheran x Nipponbare crossing
program for high salinity tolerance. In SiNas
Proceedings 2012.
Shinozaki, K., Yamaguchi-Shinozaki, K. 1997. Gene
expression and signal transduction in water stress
response. Plant Physiology, 115: 327-334.
Sudarmi. 2013. The role of molecular biology in plant
breeding. Magistra, 84 (XXV): 75-80.
Sun, W., Deng, D., Yang, L., Zheng, X., Yu, J., Pan, H.,
Zhuge, Q. 2013. Overexpression of the chloride
channel gene (GmCLC1) from soybean increases salt
tolerance in transgenic Populus deltoides × P.
euramericana 'Nanlin895'. Omics Plant Journal, 6 (5):
347-354
Sutoro, A., Bari, Subandi, Yahya, S. 2006. The genetic
parameters of Bisma corn population on different
fertilization. Variety of additives-dominant corn seed
weight. AgroBiogen Journal, 2 (2): 60-67.
Swasono, F. D. H., 2012. The role of ABA and proline in
the mechanism of adaptation of shallots to drought
stress in coastal sand soil. AgriSains Journal, 4 (5): 71-
78.
Turan, S., Cornish, K., Kumar, S. 2012. Salinity tolerance
in plants: Breeding and genetic engineering. Australian
Journal of Crop Science, 6 (9): 1337-1348
Zhang, G., Chen, M., Li, L., Xu, Z., Chen, X., Guo, J., Ma,
Y. 2009. The soybean GmERF3 overexpression of the
gene, an AP2 / ERF type transcription factor for
Increased tolerances to salt, Drought, and diseases in
transgenic tobacco. Journal of Experimental Botany, 60
(13): 3781-3796.
Evaluation of Resistance Improvement of Soybean (Glycine Max (L) Merr.) against Salinity using Mass Selection and Gene Expression of
Salinity Tolerant
37