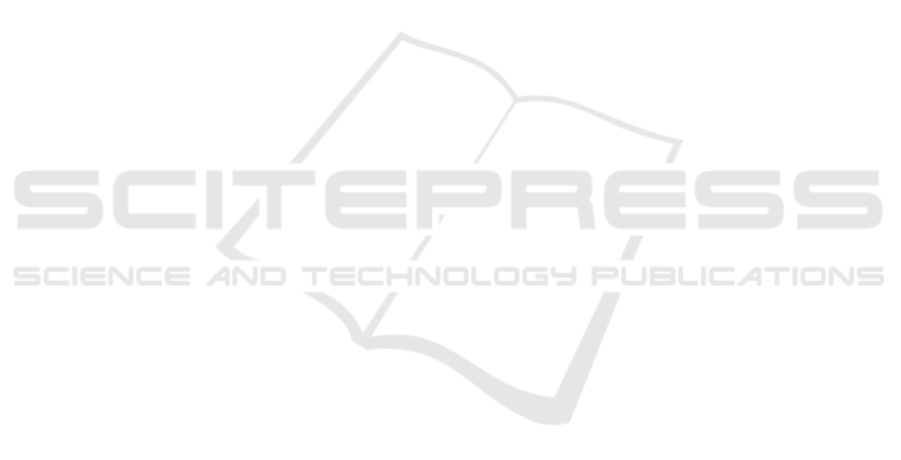
Basyuni, M., Oku, H., Baba, S., Takara, K. and Iwasaki, H.,
2007. Isoprenoids of Okinawan mangroves as lipid
input into estuarine ecosystem. Journal of
Oceanography, 63(4), 601-608.
Basyuni, M., Baba, S., Inafuku, M., Iwasaki, H., Kinjo, K.,
and Oku, H. 2009. Expression of terpenoid synthase
mRNA and terpenoid content in salt stressed mangrove.
Journal of Plant Physiology, 166(16), 1786-1800.
Basyuni, M., Baba, S., Kinjo, Y., Putri, L. A., Hakim, L.
and Oku, H., 2012. Salt-dependent increase in
triterpenoids is reversible upon transfer to fresh water
in mangrove plants Kandelia candel and Bruguiera
gymnorrhiza. Journal of Plant Physiology, 169(18),
1903-1908.
Illian, D. N., Basyuni, M., Wati, R., & Hasibuan, P. A. Z.
2018. Polyisoprenoids from Avicennia marina and
Avicennia lanata inhibit WiDr cells proliferation.
Pharmacognosy Magazine, 14(58), 513-518.
Jang, D. S., Cuendet, M., Pawlus, A. D., Kardono, L. B. S.,
Kawanishi, K., Farnsworth, N. R., Fong, H. H. S.,
Pezzuto, J. M., Kinghorn, A. D., 2004. Potential cancer
chemopreventive constituents of the leaves of
Macaranga triloba. Phytochemistry 65, 345-350.
Killops, S. D., Frewin, N. L., 1994. Triterpenoid diagenesis
and cuticular preservation. Organic Geochemistry 21,
1193-1209.
Kim, D. K., Lim, J. P., Kim, J. W., Park, H. W. and Eun,
J.S., 2005. Antitumor and antiinflammatory
constituents fromceltis sinensis. Archives of
Pharmacal Research, 28(1), 39-43.
Melo, C. M., Morais, T. C., Tomé, A. R., Brito, G. A. C.,
Chaves, M. H., Rao, V. S., & Santos, F. A. 2011. Anti-
inflammatory effect of α, β-amyrin, a triterpene from
Protium heptaphyllum, on cerulein-induced acute
pancreatitis in mice. Inflammation research, 60(7),
673-681.
Oku, H., Baba, S., Koga, H., Takara, K., and Iwasaki, H.
2003. Lipid composition of mangrove and its relevance
to salt tolerance. Journal of Plant Research, 116(1), 37-
45.
Oliveira, F. A., Chaves, M. H., Almeida, F. R. C., Lima Jr.,
R. C. P., Silva, R. M., Maia, J. L., Brito, G. A. A. C.,
Santos, F. A. and Rao, V. S., 2005. Protective effect of
- and -amyrin, a triterpene mixture from Protium
heptaphyllum (Aubl.) March. trunk wood resin, against
acetaminophen-induced liver injury in mice. Journal of
Ethnopharmacology 98, 103-108.
Sari, D. P., Basyuni, M., Hasibuan, P. A., Sumardi, S.,
Nuryawan, A. and Wati, R., 2018a. Cytotoxic and
Antiproliferative Activity of Polyisoprenoids in
Seventeen Mangroves Species Against WiDr Colon
Cancer Cells. Asian Pacific Journal of Cancer
Prevention, 19 (12), 3393-3400.
Sari, D. P., Basyuni, M., Hasibuan, P. A. Z., Wati, R., and
Sumardi. 2018b. Cytotoxic effect of polyisoprenoids
from Rhizophora mucronata and Ceriops tagal leaves
against WiDr colon cancer cell lines. Sains Malaysiana,
47(9), 1953-1959.
Sudhahar, V., Kumar, S. A., Sudharsan, P. T. and
Varalakshmi, P., 2007. Protective effect of lupeol and
its ester on cardiac abnormalities in experimental
hypercholesterolemia. Vascular Pharmacology, 46(6),
412-418.
Sumardi, S., Basyuni, M. and Wati, R., 2018. Antimicrobial
activity of polyisoprenoids of sixteen mangrove species
from North Sumatra, Indonesia. Biodiversitas Journal
of Biological Diversity, 19(4), pp.1243-1248.
Thimmappa, R., Geisler, K., Louveau, T., O'Maille, P., and
Osbourn, A. 2014. Triterpene biosynthesis in plants.
Annual Review of Plant Biology, 65, 225-257.
Versteegh, G. J., Schefuß, E., Dupont, L., Marret, F.,
Damsté, J. S. S. and Jansen, J. F., 2004. Taraxerol and
Rhizophora pollen as proxies for tracking past
mangrove ecosystems. Geochimica et Cosmochimica
Acta, 68(3), 411-422.
Volkman, J. K., 2005. Sterols and other triterpenoids:
source specificity and evolution of biosynthetic
pathways. Organic Geochemistry, 36(2),139-159.
Williams, L. A. D., 1999. Rhizophora mangle
(Rhizophoraceae) triterpenoids with insecticidal
activity. Naturwissenschaften 86, 450-452.
Yendo, A. C., de Costa, F., Gosmann, G., and Fett-Neto, A.
G. 2010. Production of plant bioactive triterpenoid
saponins: elicitation strategies and target genes to
improve yields. Molecular Biotechnology, 46(1), 94-
104.
ICONART 2019 - International Conference on Natural Resources and Technology
54