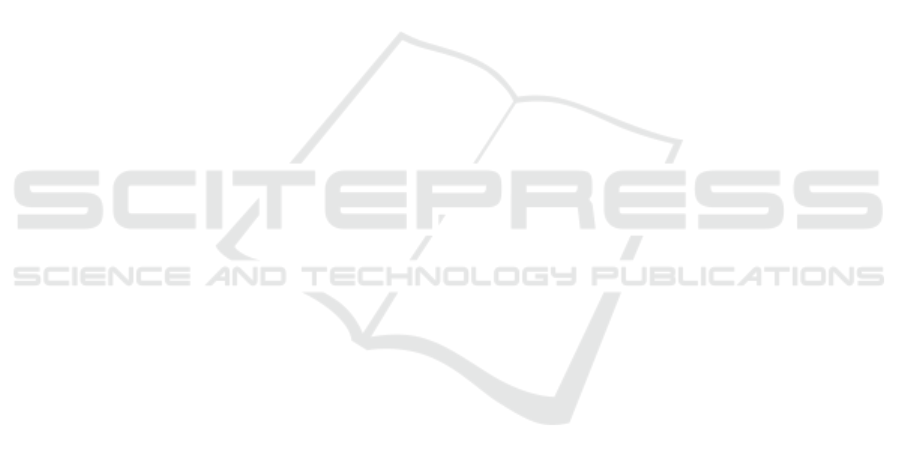
Sleiman, M., Vildozo, D., Ferronato, C., Chovelon, J.M.,
2007. Photocatalytic degradation of azo dye
metanilyellow optimization and kinetic modeling
using a chemometric approach. Applied Catalysis B.
vol. 77, no. 1-2, pp. 1–11.
Lee, Y.H., Pavlostathis, S.G., 2004. Decolorization and
toxicity of reactive anthraquinone textile dyes under
methanogenic conditions. Water Research., vol. 38,
no. 7, pp. 1838–1852.
Konstantinou, I.K., Albanis, T. A., 2004. TiO
2
-assisted
photocatalytic degradation of azo dyes in aqueous
solution: kinetic and mechanistic investigations: a
review. Applied Catalysis B. vol. 49, no. 1, pp. 1–14.
Galindo, C., Jacques, P., Kalt, A., 2001. Photooxidation of
the phenylazonaphthol AO
2
0 on TiO
2
: kinetic and
mechanistic investigations. Chemosphere. vol. 45, no.
6-7, pp. 997–1005.
Chakrabarti, S., Dutta, B.K., 2004. Photocatalytic
degradation of model textile dyes in wastewater using
ZnO as semiconductor catalyst. Journal of Hazardous
Materials. vol. 112, no. 3, pp. 269-278.
Mohabansi, N.P., Satone, A.K., 2015. Solar Photocatalytic
Degradation of Textile Effluents by Using Titanium
Dioxide. International Journal of Pharmaceutical,
Chemical & Biological Sciences.vol. 5, no. 2, pp. 487–
490.
Neppolian, B., Choi, H.C., Sakthivel, S., Arabindoo, B.,
Murugesan, V., 2002. Solar light induced and TiO
2
assisted degradation of textile dye reactive blue 4.
Chemosphere, vol. 46, no. 1, pp. 1173-1181.
Santhosh, A.M., Yogendra, K., Mahadevan, K.M.,
Madhusudhana, N., 2017. Photodegradation of Congo
red azo dye, a Carcinogenic Textile dye by using
synthesized Nickel Calciate Nanoparticles.
International Journal of Advance Research in Science
and Engineering. vol. 6, no. 7, pp. 51-64.
Madhusudhana, N., Yogendra, K., Mahadevan, K.M.,
2015. A study on photocatalytic decolourization of
Violet GL2B azo dye using two different synthesized
nano particles CaZnO
2
against commercially available
TiO
2
. International Journal of Chemical and
Environmental Engineering. vol. 6, no. 4, pp. 210-218.
Khanmohammadi, H., Rezaeian, D.K., 2015. Solar-light
induced photodecolorization of water soluble azo-
azomethine dye : influence of operational parameters
and nanophotocatalysts. Desalination and Water
Treatment.vol. 55, no. 3, pp. 655-663.
La, J., Huang, Y., Luo, G., Lai, J., Liu, C., Chu, G., 2013.
Synthesis of Bismuth Oxide Nanoparticles by Solution
Combustion Method. Particulate Science and
Technology.Vol. 31, no. 1, pp. 287-290.
Madhusudhana, N., Yogendra, K., Mahadevan, K.M.,
Santhosh, A.M., 2018. Study on Photocatalytic
Degradation Coralene Red F3BS by using Synthesized
Al
2
O
3
Nanoparticles. AGU International Journal of
Engineering and Technology.vol. 6, no. 1, pp. 29-37.
Subramani, A.K., Byrappa, K., Ananda, S., Lokanatha
Rai, K.M., Ranganathaiah, C., Yoshimura, M., 2007.
Photocatalytic degradation of indigo carmine dye
using TiO
2
impregnated activated carbon. Bulletin of
materials science.vol. 30, no. 1, pp. 37-41.
Guillard, C., Lachheb, H., Houas, A., Ksibi, M., Elaloui
E., Herrman, J.M., 2003. Influence of chemical
structure of dyes of pH and of inorganic salts on their
phtocatlytic degradation by TiO
2
Comparison of
efficiency of powder and supported TiO
2
. Journal of
Photochemistry and Photobiology A: Chemistry.vol.
158, no. 1, pp. 27-36.
Mehta, R., Surana, M., 2012. Comparative study of photo-
degradation of dye Acid Orange-8 by Fenton reagent
and Titanium Oxide- A review. Der Pharma
Chemica.vol. 4, no. 1, pp. 311-319.
Sobana, N., Thirumalai, K., Swaminathan, M., 2016.
Kinetics of Solar Light Assisted Degradation of Direct
Red 23 on Activated Carbon-loaded Zinc Oxide and
Influence of Operational Parameters. Canadian
Chemical transactions.vol. 4, no. 1, pp. 77-89.
Sakthivel, S., Neppolian, B., Shankar, M.V., Arabindoo,
B., Palanichamy, M., Murugesan, V., 2003. Solar
photocatalytic degradation of azo dye: comparison of
photocatalytic efficiency of ZnO and TiO
2
. Solar
Energy Materials & Solar Cells.vol. 77, no. 1, pp. 65–
82.
Gopalappa, H., Yogendra, K., Mahadevan, K.M.,
Madhusudhana, N., 2012. Solar Photocatalytic
Degradation of Orange G (Mono azo dye) and C.I.
Direct Yellow 50 (Di azo dye) by Synthesized
CaZnO
2
Nanoparticle in aqueous solution.
International Journal of Universal Pharmacy and Life
Sciences.vol. 2, no. 4, pp. 66-77.
Mirkhani, V., Tangestaninejad, S., Moghadam, M.,
Habibi, M.H., Rostami, V.A., 2009. Photocatalytic
Degradation of Azo dyes Catalyzed by Ag Doped
TiO
2
Photocatalyst. Journal of Iranian Chemical
Society.vol. 6, no. 3, pp. 578-587.
Byrappa, K., Subramani, A.K., Ananda, S., LokanathaRai,
K.M., Dinesh, R., Yoshimura, M., 2006.
Photocatalytic degradation of Rhodamine B dye using
hydrothermally synthesized ZnO. Bulletin of Material
Sciences.vol. 29, no. 5, pp. 433-438.
Guillen, S.A., Mayen, S.A., Torres-Delgado, G.,
Castanedo-Perez, R., Maldonado, A., De la Olvera,
M.L., 2010. Photocatalytic degradation of methylene
blue using undoped and Ag doped TiO
2
thin films
deposited by a sol gel process: Effect of the ageing
time of the starting solution and the film thickness.
Materials Science and Engineering: B.vol. 174, no. 1-
3, pp. 84-87.
Movahedi, M., Mahjoub, A.R., Janitabar-Darzi, S., 2009.
Photodegradation of Congo Red in Aqueous Solution
on ZnO as an Alternative Catalyst to TiO
2
. Journal of
the Iranian Chemical Society.vol. 6, no. 3, pp. 570-
577.
ICFEPP 2019 - International conference on Future Environment Pollution and Prevention
16