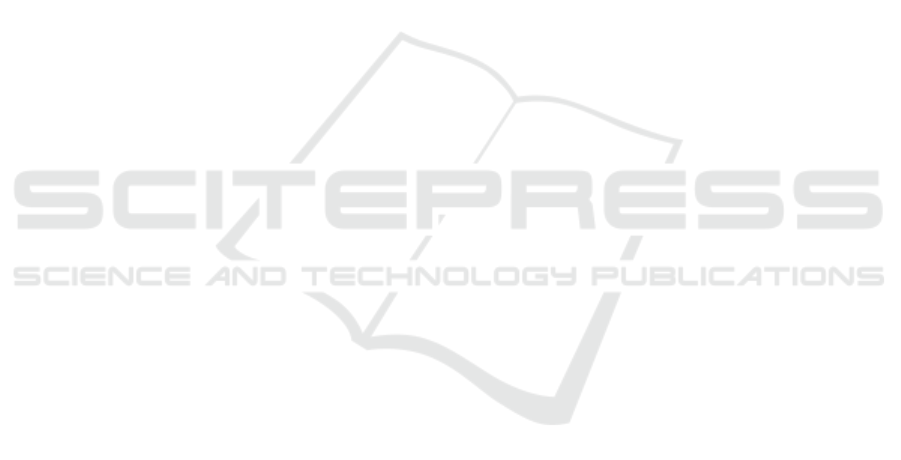
predict. There are not much known about the
gasification characteristics of non-lignocellulosic
biomass which has low or no cellulose,
hemicellulose and lignin content. Protein, which is
non-lignocellulosic, is a common important
component of biomass, organic waste, food waste,
animal matter, and algae which are usually wet
biomass. Then, protein gasification characteristics is
important to study that its molecule is different from
carbohydrate and has N-containing. Amino acids
are good representative model compounds of protein
due to they are produced by the hydrolysis of
protein. So far, glycine, alanine, valine, leucine, and
proline have been studied their gasification
characteristics (Samanmulya et al., 2014). These
employed amino acids showed the different
gasification behavior but only the gasification rate of
glycine and alanine are practically identical, and
they were considered to be a standard for
determining amino acid gasification. By the
different stability of the bond between the carboxyl
group and amino group and functional group, the
other three amino acids behave variously from the
standard amino acids and the different sensitivity of
the produced radicals also affect to their gasification
characteristics. Then, more gasification
characteristics data is required to be able to predict
the gasification characteristics of amino acids.
Moreover, the correlation between kinetic rate and
temperature are useful to hypothesize and
understand the reaction mechanism of supercritical
water gasification of biomass (Promdej and
Matsumura, 2011). The reactions between
intermediates were differentiated to be radical and
ionic reactions by their compatibility to Arrhenius
behavior (Yong and Matsumura, 2013). Therefore,
Arrhenius rate law has attracted attention to
determine supercritical water gasification
characteristics of amino acids.
Glutamic acid and arginine are of interest
because they are mostly found in a variety of foods
including animal source and plant source. They are
classified in different category which are acidic and
basic, respectively, and moreover, their gasification
characteristics have not much been studied yet.
This study purpose is to determine the effect of two
carboxyl groups, guanidine functional group, acidity
and alkalinity on supercritical water gasification.
Furthermore, the obtained reaction products were
evaluated based on qualitative and quantitative, and
the kinetics parameters were also elucidated for the
reaction rate of glutamic acid and arginine
gasification.
2 EXPERIMENTAL SECTION
2.1 Experimental Procedure
All gasification experiments were performed using
the tubular flow reactor which was schematically
illustrated in our previous study (Samanmulya et al.,
2014). Briefly, a SS316 steel tube with a length of
12 m and an inner diameter of 2.17mm was used as
the reactor. The reaction temperature was varied
from 500 to 650
o
C (residence times in a range of
86–119 s) and reaction pressure was fixed at 25
MPa. Before the addition of the feedstock, the
reactor pressure was maintained at 25 MPa by
feeding only deionized water and controlled by
back-pressure regulator, and the reactor temperature
was reached the desired temperature. Glutamic acid
and arginine, obtained from Nacalai Tesque with
purity >98%, aqueous solutions of 1.0 wt% were
prepared by dilution in deionized water and fed into
the reactor at a feedstock flow rate of 2 g/min. After
passing through the reactor, the effluent was cooled
down in a heat exchanger, depressurized by a back-
pressure regulator, and then sampled.
2.2 Analytical Methods
The rate of gas generation was measured using a
water displacement method in which we measured
the time required for the effluent gas to fill a vial of
known volume. The gaseous product was
characterized and quantified using gas
chromatography (GC). Carbon dioxide and carbon
monoxide were detected by GC with a thermal
conductivity detector (GC-TCD) using helium as the
carrier gas. Methane, ethene, and ethane were
detected using GC with a flame ionization detector
(GC-FID) using helium as the carrier gas. Hydrogen
was detected by GC-TCD with nitrogen as the
carrier gas.
The liquid product was quantified the amounts of
carbon in the liquid product (non-purgeable organic
carbon, NPOC) and the dissolved carbon gas
product (inorganic carbon, IC) by a total organic
carbon (TOC) analyzer.
Although, gasification of nitrogen contained
molecule that can produce syngas with NOx but N-
containing molecule is finally converted to N
2
via
intermediate compound of NH
3
generation in
supercritical water gasification system and no NOx
is occurred (Goto et al., 1998 and Yakaboylu et al.,
2013).
ICFEPP 2019 - International conference on Future Environment Pollution and Prevention
18