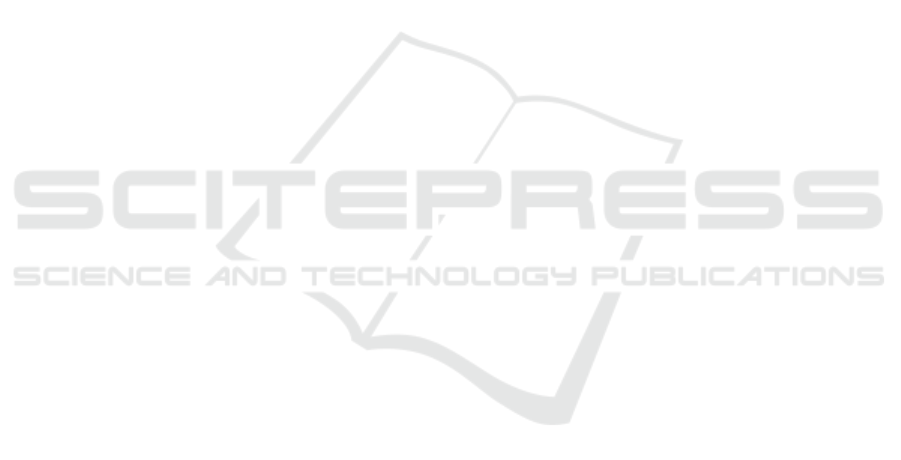
Aqueous Solution onto Zeolites from Coal Fly and
Bottom Ashes. Orbital: Electron. J. Chem. 5 (3):179-
191.
Bronislaw Buczek., 2016. Preparation of Active Carbon by
Additional Activation with Potassium Hydroxide and
Characterization of Their Properties. Advances in
Materials Science and Engineering, Volume 2016,
Article ID 5819208, 4 pages.
Charles, Osu., and Odoemelam, S.A., 2010. Study on
Adsorbent Dosage, Particle Sizes and Ph Constraints on
Biosorption of Pb (II) and Cd (II) Ions from Aqueous
Solution Using Modified and Unmodified Crasstrotrea
Gasar (Bivalve) Biomass. International Archive of
Applied Sciences and Technology, Vol. 1 (1) June
2010: 62-68 (2010).
Cordell, D., Drangert, J. O., White, S., 2009. The Story of
Phosphorus: Global Food Security and Food for
Thought. Global Environmental Change 19 (2009)
292–305.
Dincer, A.R., Gunes¸ Y., Karakaya, N., 2007. Coal-based
Bottom Ash (CBBA) Waste Material as Adsorbent for
Removal of Textile Dyestuffs from Aqueous Solution.
Journal of Hazardous Materials 141 529–535.
Freundlich, H. M. F., 1906. Uber die Adsorption in
Losungen. Zeithschrift fur Physikalische Chemie
(Leipzig). 57A, 385-470.
Gandhimathi, R., Ramesh, S.T., Sindhu, V., Nidheesh,
P.V., 2013.. Bottom ash Adsorption of Basic Dyes from
Their Binary Aqueous Solutions. Songklanakarin J. Sci.
Technol. 35 (3), 339-347.
Ghaneian, M.T., Ghanizadeh, G., Alizadeh, M.T.H.,
Ehrampoush, M.H., Said, F.M., 2014. Equilibrium and
Kinetics of Phosphorous Adsorption onto Bone
Charcoal from Aqueous Solution. Environ Technol. 35
882-890.
Gorme, J.B., Maniquiz, M.C., Kim, S.S., Son, Y.G., Kim,
Y.T., Kim, L.H., 2010. Characterization of Bottom Ash
as an Adsorbent of Lead from Aqueous Solutions.
Environ. Eng. Res.15(4) : 207-213.
Hasfalina, Man, C., Chin, W.H., Zadeh, M.R., Rashid, M.,
Yusof., M., 2012. Adsorption Potential of Unmodified
Rice Husk for Boron Removal. Bioresources 7 (3),
3810-3822.
Ikenyiri PN., Ukpaka CP., 2016. Overview on the Effect of
Particle Size on the Performance of Wood Based
Adsorbent. J Chem Eng Process Technol 7: 315. doi:
10.4172/2157-7048.1000315.
Kumar, P.S., Korving, L., Keesman, K.J., M.C.M. van
Loosdrecht, M.CM., 2019. Eff ect of Pore Size
Distribution and Particle Size of Porous Metal Oxides
on Phosphate Adsorption Capacity and Kinetics.
Chemical Engineering Journal 358 (2019) 160–169.
Ma J., Sun Y., Yu F., 2017. Efficient Removal of
Tetracycline with KOH-Activated Graphene from
Aqueous Solution. R. Soc. open sci. 4: 170731.
Mittal, J., Jhare, D., Vardhan, H., Mittal, A., 2013.
Desalination and Water Treatment : Utilization of
Bottom Ash as a Low-Cost Sorbent for the Removal
and Recovery of a Toxic Halogen Containing Dye
Eosin Yellow. Desalination and Water Treatment, DOI:
10.1080/19443994.2013.803265.
Muhmood, A., Wu, S., Lu, J., Ajmal, Z., Luo, H., Dong,
R., 2018. Nutrient Recovery from Anaerobically
Digested Chicken Slurry via Struvite: Performance
Optimization and Interactions with Heavy Metals and
Pathogens. Sci. Total Environ. 635 1-9.
Onar, A. Nur, Balkava, N., Akyuz. T., 1996. Phosphate
Removal by Adsorption. Enviromental Technology,
Vol. 17 pp 207-2013, Publications Division Selper Ltd.
Peleka, E.N., Mavros, P.P., Zamboulis, D., Matis, K.A.,
2006. Removal of Phosphates from Water by a Hybrid
Flotation–Membrane Filtration Cell. Desalination 198
198-207.
Peng, Y.Z., Wang, X.L., Li, B.K., 2006. Anoxic Biological
Phosphorus Uptake and the Effect of Excessive
Aeration On Biological Phosphorus Removal In The
A(2)O Process. Desalination 189(1-3) 155-164.
Saleh, S.M., Maarof, H.I., Rahim, S.N.S.A., Nasuha, N.,
2012.. Adsorption Congo Red into Bottom Ash. Journal
Of Applied Sciences 12 (11): 1181-1185.
Seftel, E.M., Ciocarlan, R.G., Michielsen, Meynen, V.,
Mullens, S., Cool, P., 2018. Insights into Phosphate
Adsorption Behavior on Structurally Modified ZnAl
Layered Double Hydroxides. Applied Clay Science 165
234–246.
Siwek, Hanna, Artur Bartkowiak, and Malgorzata
Wlodarczyk., 2019. Adsorption of Phosphates from
Aqueous Solutions on Alginate/Goethite Hydrogel
Composite. Water 2019, 11, 633;
doi:10.3390/w11040633.
Usman, M., Byrne, J.M., Chaudhary, A., Orsetti, S., Hanna,
K., Ruby, C., Kappler, A., Haderlein, S.B., 2018.
Magnetite and Green Rust: Synthesis, Properties, and
Environmental Applications of Mixed-Valent Iron
Minerals. Chem. Rev. 118(7) 3251-3304.
Van der Houwen, J., Valsami-Jones, E., 2001. The
Application of Calcium Phosphate Precipitation
Chemistry to Phosphorus Recovery: The Influence of
Organic Ligands Environ. Technol. 22 1325-1335.
Waseem, S., Din, M.I., Naseer, S.,. Rasool, A., 2012.
Evaluation of Acacia Nilotica as a Non Conventional
Low Cost Biosorbent for the Elimination of Pb (II) and
Cd (II) Ions from Aqueous Solutions. Arabian Journal
of Chemistry, VII.
ICOCSTI 2019 - International Conference on Chemical Science and Technology Innovation
104