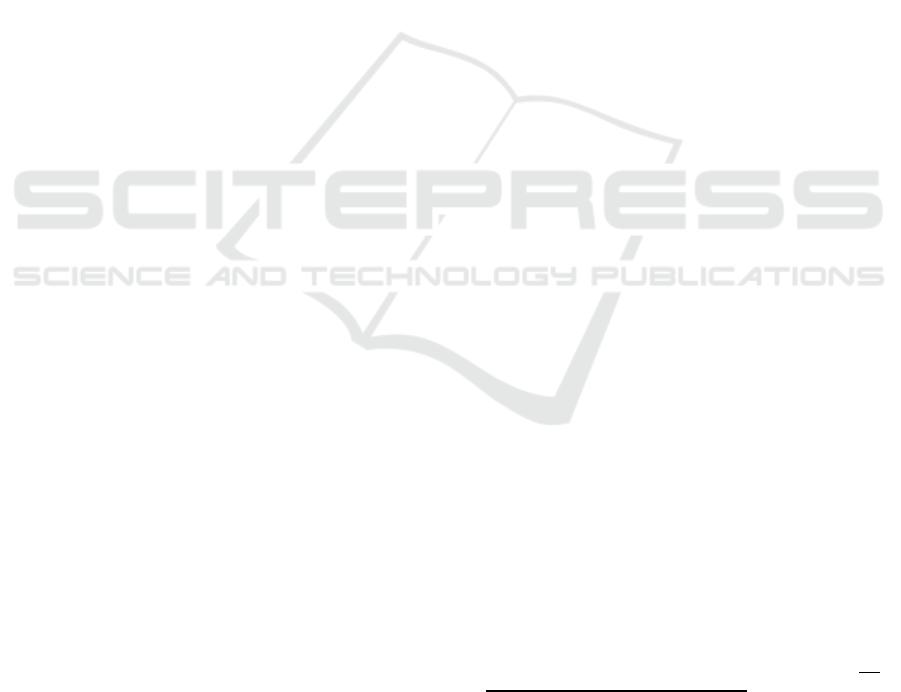
electromagnetic wave on the basis of GPS. By
measuring the time difference of arrival (TDOA) of
two interrelated entangled light beams, the distance
between satellite and user and the spatial coordinates
of user are calculated according to the acquired
TDOA. The satellite-based interferometric QPS
proposed by the US Army Research Laboratory,
combined with the traditional satellite positioning
idea and the optical quantum entanglement pulse
interferometric ranging technology, is the first to put
forward the design scheme of the satellite-based
QPS to achieve positioning. It uses satellite to
broadcast quantum signals. The basic structure of
QPS is composed of three baselines which are
composed of two groups of satellites with six known
positions. The satellites are located in two focal
points of a hyperboloid, and the user is at the
intersection point of a hyperboloid consisting of a
hyperboloid. But the disadvantage is that six
satellites with three baselines are needed to
participate in positioning, which is costly.
(Giovannetti, & V, 2004)
Based on the existing active QPS, this paper
improves the implementation scheme of baseline
interferometric quantum positioning system
proposed by Dr. Thomas B. Bahder, U. S. Army
Research Laboratory. An improved QPS based on
three satellites is proposed to locate users. The
number of satellites needed and the complexity of
the system is reduced. The whole process of ranging
and positioning of the improved satellite-based QPS
is analyzed and the system structure is designed.
Firstly, a satellite-ground optical link is established
between the satellite and the ground. The quantum
entangled light emitted by the user equipment and
transmits to the satellite along the satellite-ground
optical link, and then returns to the user through the
reflection of the satellite along the original path. The
TDOA of signal light and reference light is obtained
by pulse matching counting and data fitting with
least square method. Finally, the distance and user
space coordinates are calculated according to the
TDOA simultaneous equations obtained from
entangled light of three satellites. Through the
Acquisition Tracking and Pointing (ATP) system to
achieve the uninterrupted positioning of the user.
The structure of this paper is as follows: Firstly, the
flow of the whole system is explained, including the
establishment of satellite-ground optical link, the
acquisition of TDOA by entangled light, and then
ranging and location solution by simultaneous
equations. (Bahder, T. B, 2004)
2 BASIC PRINCIPLE OF
IMPROVED SATELLITE-
BASED QPS LOCATION
The ranging and positioning process of improved
satellite-based QPS can be divided into three parts:
Firstly, a stable optical link is established between
satellites and the earth, and the positioning are
carried out by using quantum entangled optical
dynamic communication. The establishment of
satellite-to-ground optical link is to provide accurate
optical links for quantum entangled optical signals to
propagate between satellites and users, including
beacon light emission, acquisition, tracking and
aiming. These four sub-processes are realized by
ATP system. Then, based on the quantum entangled
light ranging, according to the established satellite-
ground optical link, the quantum entangled light
dynamic communication is used for ranging and
positioning. Its working process is divided into the
emission and reception of quantum entangled light
and the acquisition of TDOA. Finally, the position is
calculated based on the equations obtained by
TDOA quantum ranging. (Yang, C, et.al, 2010)
Geometric positioning principle is used in
positioning calculation. Two entangled beams of
light emitted by the entangled photon pairs generator
at the user end, one of which arrives at the satellite
along the satellite-ground optical link and reflects
back to the ground from the satellite, then received
by a single photon detector on the user side; the
other light is directly emitted to another single
photon detector on the user side to complete the
emission and reception of entangled photon pairs.
The distance is calculated by using TDOA of two
entangled beams. The user's spatial coordinates are
calculated by three TDOAs obtained from three
satellites.
When the difference of arrival time
between
satellite n (n=1, 2, 3) and user is obtained, the
distance between satellite and user can be calculated
as
. Let the space coordinates of
three quantum satellites be
and
respectively. The formula of
the relationship between the distance between each
satellite and the user and the coordinates of the
ground user can be obtained:
. By measuring
the arrival time difference of entangled light from
the user to three satellites, three formulas of parallel
equations are obtained:
Research of An Improved Satellite-based Quantum Positioning System
457