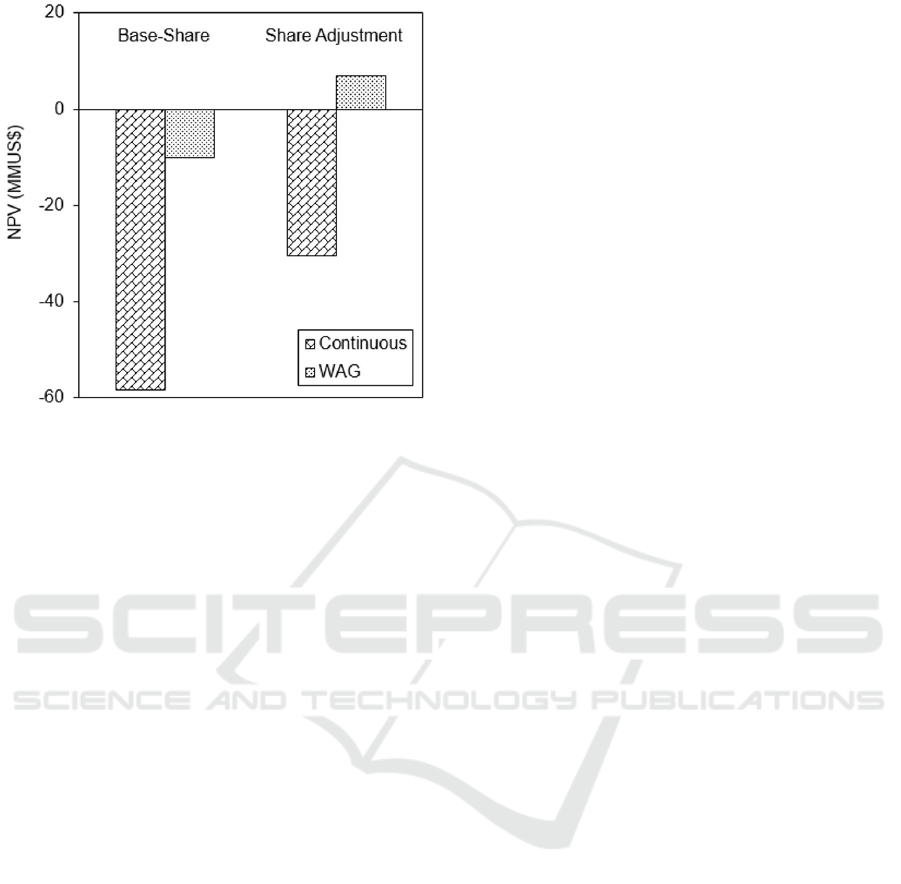
Figure 9: Effect of share adjustment to NPV for continuous
and WAG CO
2
flooding.
4 CONCLUSIONS
This paper analyze the feasibility of CO
2
project
under Indonesia gross split mechanism by using
reservoir simulation method. One of Indonesia oil
field reservoir is modelled for this study, where the
CO
2
injection schemes is limited to continuous and
WAG scenarios. Simulation results reveals a better
performance of WAG in recovering remaining oil in
the reservoir. Moreover, feasible indication is shown
on WAG scheme after adjusting the base share of
government and contractor. Including the CO
2
issues
into the variable and progressive share, points may
increase the tendency of CO
2
flooding application in
Indonesia
REFERENCES
Abdurrahman, M., Permadi, A., and Bae, W. (2015). An
improved method for estimating minimum miscibility
pressure through condensation–extraction process
under swelling tests. Journal of Petroleum Science
and Engineering, 131:165–171.
Abedini, A. and Torabi, F. (2014). Oil recovery
performance of immiscible and miscible co2
huff-and-puff processes. Energy & Fuels,
28(2):774–784.
Agency, I. E. (2016). 20 Years of Carbon Capture
and Storage: Accelerating Future Deployment.
International Energy Agency.
Azzolina, N. A., Nakles, D. V., Gorecki, C. D., Peck,
W. D., Ayash, S. C., Melzer, L. S., and Chatterjee,
S. (2015). Co2 storage associated with co2 enhanced
oil recovery: A statistical analysis of historical
operations. International Journal of Greenhouse Gas
Control, 37:384–397.
Christensen, J. R., Stenby, E. H., Skauge, A., et al.
(1998). Review of wag field experience. In
International petroleum conference and exhibition of
Mexico. Society of Petroleum Engineers.
Giranza, M. and Bergman, A. (2018). Indonesia’s new
gross split psc: Is it more superior than the previous
standard psc. Journal of Economics, Business and
Management, 6.
Irham, S. and Julyus, P. (2018). The new energy
management policy: Indonesian psc-gross-split
applied on steam flooding project. In IOP Conference
Series: Earth and Environmental Science, volume
106, page 012109. IOP Publishing.
Jaafar, M., Omar, S., Anuar, S., and Suradi, S.
(2014). Reservoir monitoring of eor processes (wag,
foam and polymer) using streaming potential. In
Scientific Cooperations International Workshops on
Engineering Branches, pages 8–9.
Jarrell, P. M., Fox, C. E., Stein, M. H., and Webb, S. L.
(2002). Practical aspects of CO2 flooding, volume 22.
Society of Petroleum Engineers Richardson, TX.
Jishun, Q., Haishui, H., and Xiaolei, L. (2015). Application
and enlightenment of carbon dioxide flooding in the
united states of america. Petroleum Exploration and
Development, 42(2):232–240.
Roach, B. and Dunstan, A. (2018). The indonesian psc: the
end of an era. The Journal of World Energy Law &
Business, 11(2):116–135.
Rubin, E. S., Davison, J. E., and Herzog, H. J. (2015). The
cost of co2 capture and storage. International Journal
of Greenhouse Gas Control, 40:378–400.
Whittaker, S., Rostron, B., Hawkes, C., Gardner, C., White,
D., Johnson, J., Chalaturnyk, R., and Seeburger, D.
(2011). A decade of co2 injection into depleting
oil fields: Monitoring and research activities of the
iea ghg weyburn-midale co2 monitoring and storage
project. Energy Procedia, 4:6069–6076.
Feasibility Study of CO2 Flooding under Gross-split Mechanism: Simulation Approach
19