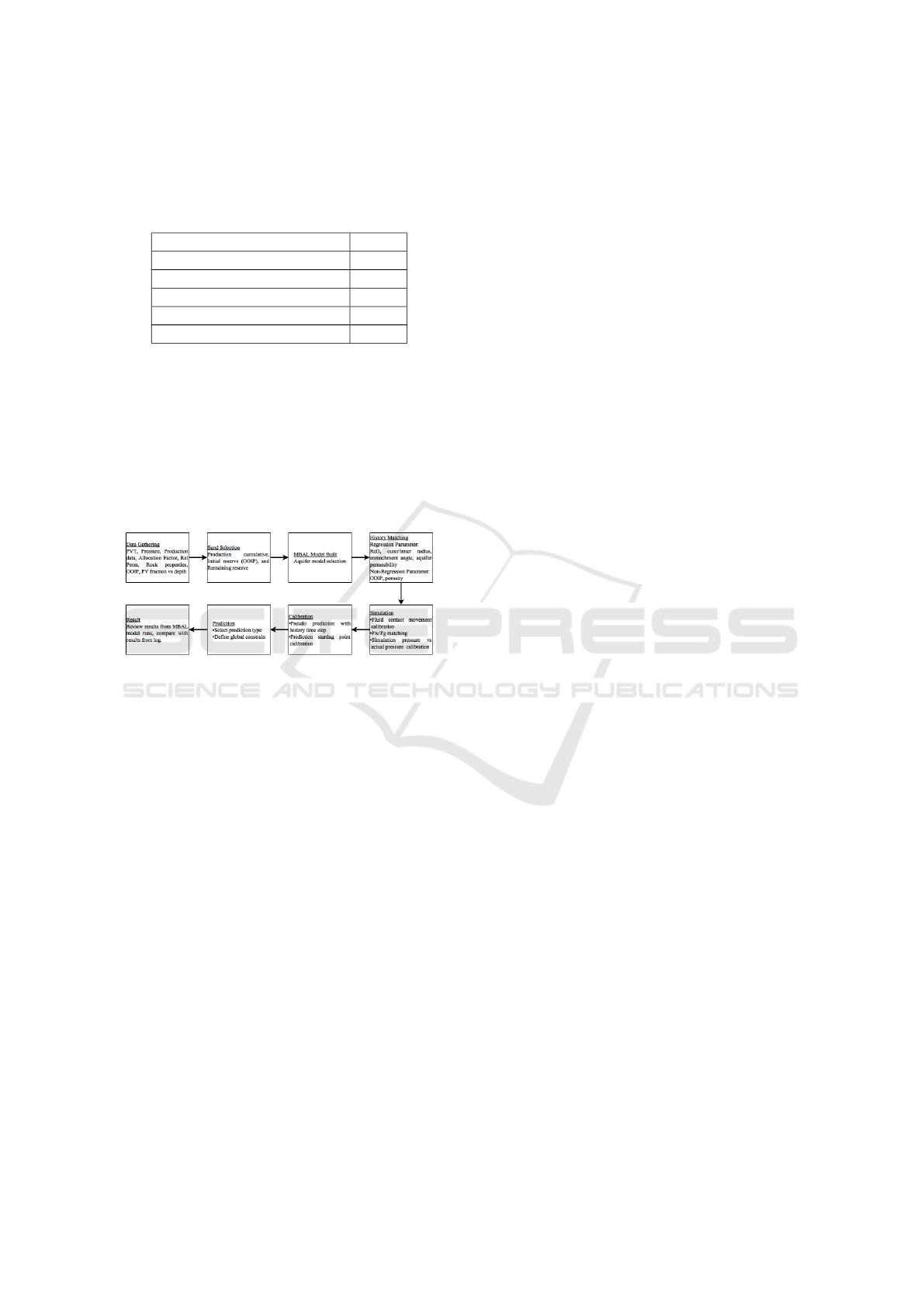
WH field reservoir properties from the log data,
core, single well-tracer, and volumetric data are as
follows:
Table 1: WH Field Reservoir Properties
Formation GOR, SCF/STB 26.4
Oil Gravity, API 34.5
Gas Gravity, sp. Gravity 0.8
Water Salinity, ppm 20000
Connate Water Saturation, % 21
Porosity, % 25
3 METHODOLOGY
In this section, the methodology, which applied in
this paper will be discussed in order to build the sand
predictive material balance equation models by using
IPM – MBAL software.
Figure 2: General IPM - MBAL Workflow
3.1 Data Gathering
Proper data acquisition has to be carried out in or-
der to build a good material balance equation model
or MBAL model. Most of these data are acquired
at the early phase of field development. Either us-
ing well tests (RFT, MDT, Swab, PBU, etc) or core
test (RCAL or SCAL) data acquired are, Pressure,
Production data, PVT, Rock properties, OOIP from
the volumetric calculation, and PV fraction vs depth.
Porosity, permeability, and water connate saturated
also are obtained from existing well logs and core
data. Original oil in place (OOIP) obtained by calcu-
lating the rock properties (porosity, water connate sat-
uration, formation volume factor) and net pay thick-
ness and area from well-logs to get the OOIP math-
ematically. Effort should be made in order to under-
stand the uncertainties related to the reservoir param-
eters, which used to calculate OOIP. In cases when the
MBAL initialize volumes are different from the vol-
umetric calculated volumes, basically due to the high
uncertainty of the MBAL data which is used in the
simulation.
3.2 Sand Selection
Sand selection is needed to filter which sand is suit-
able to model and develop in the future. The screen-
ing criteria of this section initial volumetric OOIP,
production cumulative, and remaining reserves. In
this case, when the remaining reserves are too low for
a layer, it will not profit to develop. C, E, K, and L
are the selected sand based on these screening crite-
ria, which are suitable to model and develop.
3.3 Material Balance Model
The understanding of building a material balance
model for each productive layer is needed to make a
sand predictive model in material balance. It requires
basic and fundamental knowledge related to the reser-
voir structure, type, and the aquifer effect to the reser-
voir itself. Several analytical models of the aquifer
were tested in a bid to model the geometry of the
reservoir. Carter Stacy, Van Everdingen, Van Everdin-
gen modified, Hurst-Van Everdingen modified, etc
are the available aquifer models at the software. Af-
ter aquifer model selection (in this case, Hurst-Van
Everdingen modified model was selected), the model
already established to connect the reservoir volume.
The predicted OOIP which generated by the software
can be compared with the volumetric OOIP. In this
case, the generated OOIP is matched to the volumetric
OOIP for all layers (see Fig 2 for initialization model
plots).
3.4 History Matching
With the aquifer model being the key of uncertainty,
encroachment angle, ReD, aquifer permeability, and
inner/outer ratio were regressed upon the reservoir
pressure history matching process and production
data assuming reservoir volume reproduced to stock
tank condition. The regression needs to be done re-
peatedly until the deviation is lower than 5. It needs
to be done in order to validate the model due to the
aquifer model uncertainties.
3.5 Simulation
At this part, reservoir pressure over time is simu-
lated from the production history data. This simulated
reservoir pressure is compared to the measured reser-
voir pressure at the field from the input data to see
the MBAL model could replicate the actual or current
reservoir pressure which is given by the same reser-
voir energy and properties (see Fig 3 to Fig 6). Sim-
Predicting of Oil Water Contact Level using Material Balance Modeling of a Multi-tank Reservoir
333