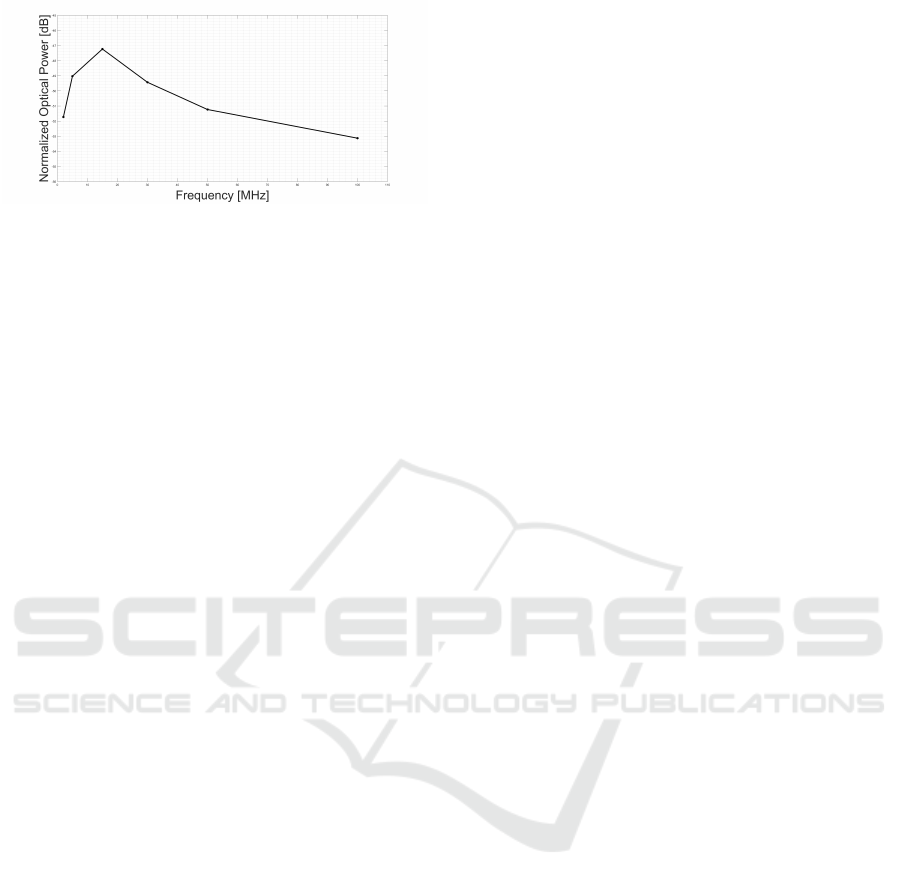
Figure 10: Normalized optical power to the bias point
against modulation frequency.
4 CONCLUSIONS
Compact and low power systems are two fundamen-
tals requirements for systems operating in particular
scenarios such as underwater communications with
mini vehicles. To that end we assessed the feasibility
of using a simple, compact, and passive copper heat
sink to control a GaN laser diode emitting around 450
nm. Actually, the junction temperature reaches a suit-
able steady-state behavior either driving the laser with
a continuous or sinusoidal waveform.
REFERENCES
Akasaki, I. (2013). Gan-based p–n junction blue-
light-emitting devices. Proceedings of the IEEE,
101(10):2200–2210.
Chi, Y.-C., Wu, T.-C., Lin, C.-Y., Lu, H.-H., Kuo, H.-
C., and Lin, G.-R. (2016). Underwater 6.4-m optical
wireless communication with 8.8-gbps encoded 450-
nm gan laser diode. In 2016 International Semicon-
ductor Laser Conference (ISLC), pages 1–2. IEEE.
Doniec, M. and Rus, D. (2010). Bidirectional optical com-
munication with aquaoptical ii. In 2010 IEEE Interna-
tional Conference on Communication Systems, pages
390–394. IEEE.
Farr, N., Bowen, A., Ware, J., Pontbriand, C., and Tivey,
M. (2010). An integrated, underwater optical/acoustic
communications system. In OCEANS’10 IEEE SYD-
NEY, pages 1–6. IEEE.
Han, S., Noh, Y., Liang, R., Chen, R., Cheng, Y.-J., and
Gerla, M. (2014). Evaluation of underwater optical-
acoustic hybrid network. China Communications,
11(5):49–59.
Hwang, W. J., Lee, T. H., Choi, J. H., Kim, H. K., Nam,
O. H., Park, Y., and Shin, M. W. (2007). Thermal in-
vestigation of gan-based laser diode package. IEEE
Transactions on components and packaging technolo-
gies, 30(4):637–642.
Karim, A. (2004). Measurement of junction temperature
of a semiconductor laser diode. In 8th International
Multitopic Conference, 2004. Proceedings of INMIC
2004., pages 659–662. IEEE.
Kaushal, H. and Kaddoum, G. (2016). Underwater optical
wireless communication. IEEE access, 4:1518–1547.
Lee, C., Zhang, C., Cantore, M., Farrell, R. M., Oh, S. H.,
Margalith, T., Speck, J. S., Nakamura, S., Bowers,
J. E., and DenBaars, S. P. (2015). 4 gbps direct mod-
ulation of 450 nm gan laser for high-speed visible
light communication. Optics express, 23(12):16232–
16237.
Lee, C. C. and Velasquez, J. (1998). Transient thermal
measurement of laser diodes. In 1998 Proceedings.
48th Electronic Components and Technology Confer-
ence (Cat. No. 98CH36206), pages 1427–1430. IEEE.
Lodovisi, C., Loreti, P., Bracciale, L., and Betti, S. (2018).
Performance analysis of hybrid optical–acoustic auv
swarms for marine monitoring. Future Internet,
10(7):65.
Moriconi, C., Cupertino, G., Betti, S., and Tabacchiera, M.
(2015). Hybrid acoustic/optic communications in un-
derwater swarms. In OCEANS 2015-Genova, pages
1–9. IEEE.
Najda, S. P., Perlin, P., Suski, T., Marona, L., Leszczy
´
nski,
M., Wisniewski, P., Czernecki, R., Kucharski, R., Tar-
gowski, G., Watson, M. A., et al. (2016). Algainn
laser diode technology for ghz high-speed visible light
communication through plastic optical fiber and water.
Optical Engineering, 55(2):026112.
Siegal, B. (2002). Laser diode junction temperature mea-
surement alternatives: An overview. In Proceedings
of PhoPack 2002.
Wu, T.-C., Chi, Y.-C., Wang, H.-Y., Tsai, C.-T., and Lin, G.-
R. (2017). Blue laser diode enables underwater com-
munication at 12.4 gbps. Scientific reports, 7:40480.
PHOTOPTICS 2020 - 8th International Conference on Photonics, Optics and Laser Technology
44